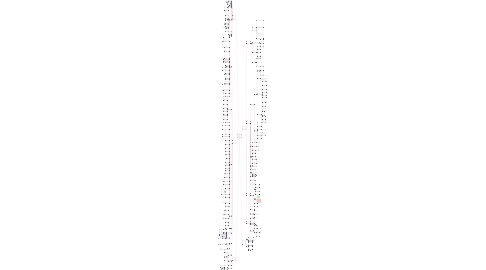
1. Control
1.1. active
1.1.1. Fast Model Predictive Control (FMPC)
1.1.2. Model Predictive Control (MPC)
1.1.3. periodic optimal control
1.2. passive
1.2.1. Pilot Kite
1.2.2. Arch/multiple anchorpoints
1.2.3. Mechanical
1.2.4. auto-zenith
2. Projects
2.1. Academic
2.1.1. ALU-FR / SYSCOP / Systems Control and Optimization Laboratory IMTEK, Faculty of Engineering, University of Freiburg
2.1.2. University of Porte: UP WIND
2.1.3. Politecnico Milano / Dipartimento di Elettronica
2.1.4. SkyPower100
2.1.5. Automatic control research group CHALMERS UNIVERSITY OF TECHNOLOGY
2.1.6. Gipsa-lab Automatic Control Department, Grenoble Institute of Technology (G-INP)
2.1.7. Research group "Control of renewable energy systems" Munich School of Engineering
2.1.8. KitePower/TU Delft
2.1.9. KU Leuven
2.1.10. University of Limerick
2.1.11. AUTOMATIC CONTROL LABORATORY EPFL
2.1.12. OMNIDEA
2.1.13. NASA Langley Research Center
2.1.14. UNC Charlotte
2.1.15. FH Muenster / Kitewiki
2.1.16. GIPSA-lab Grenoble
2.1.17. UFSCkite research group
2.1.18. Kanagawa Institute of Techology
2.1.19. WindHawk Solutions/University of Massachusetts Lowell
2.1.20. ftero / ETH Zurich
2.2. Open Source / Free Hardware
2.2.1. AWEsome
2.2.2. Robokite
2.2.3. Kite Power Coop
2.2.4. ENPH479
2.2.5. VisVentis
2.2.6. Free KiteSim
2.2.7. kPower
2.2.8. OTS on Wevolver
2.3. Commercial
2.3.1. Makani
2.3.2. Aenarete
2.3.3. Pacific Sky Power
2.3.4. kiteKRAFT
2.3.5. Altaeros Energies
2.3.6. Flygenkite
2.3.7. e-kite
2.3.8. KiteGen
2.3.9. Sky Windpower
2.3.10. SkySails
2.3.11. EnerKite
2.3.12. kitemill.no/
2.3.13. Ampyxpower
2.3.14. TwingTec
2.3.15. eWindSolutions
2.3.16. Enevate/TU Delft spin off
2.3.17. windswept-and-interesting
2.3.18. SELSAM SuperTurbine / USwindLabs
2.3.19. Windlift
2.3.20. KiteWinder
2.3.21. Skypull
2.3.22. Bladetips Energy
2.3.23. Skeiron / QConcepts
2.4. Graveyard
2.4.1. Twind
2.4.2. Crosswind Power Systems
2.4.3. airborne-wind-turbine/ATENA
2.4.4. Highest Wind LLC
2.4.5. Magenn Power
2.5. CGI
2.5.1. LTA windpower
2.6. Networks/Industry groups and Forums
2.6.1. AWESCO
2.6.2. Airborne Wind Europe
2.6.3. hwn500.de
2.6.4. bhwe.org
2.6.5. Airborne Wind Energy Consortium (AWEC)
2.6.6. Airborne Wind Energy Industry Association (AWEIA)
2.6.7. AWES Forum
2.6.8. AWES Museum / Energy Kite Systems
2.7. Components
2.7.1. Motors/Generators
2.7.1.1. Joby Motors
2.7.2. Sensors & Controls
2.7.2.1. Aenarete
2.7.3. Winches
2.7.3.1. FEcreate
3. Secondary challenges
3.1. Overspeed protection
3.2. Intermittency of wind / Stay airborne
3.3. Lightning protection
3.4. Icing
3.5. Airspace restrictions
3.6. Scalability
3.7. Visual Impact
3.8. Land&Airspace use
3.9. Launching
3.9.1. Catapult/Canon/Rocket
3.9.2. Carrier
3.9.3. Rotational
3.9.4. Pilot/Lifter kite / Kytoon
3.9.5. Ferry/drop
3.9.6. VTOL
3.9.7. Phased tugging/pumping
3.9.8. Wind generators
3.9.9. Winch acceleration&on board propulsion
3.9.10. Bungee-Launch
3.9.11. Water launch
3.10. Reliability
3.11. Turbulence
3.12. Partial failure
4. Other Design Decisions
4.1. Number of tethers
4.1.1. 0/untethered
4.1.2. 1
4.1.3. 2
4.1.4. 3
4.2. Operating altitute
4.3. Soft/Hard wing
4.4. Flightpath
4.4.1. Crosswind
4.4.1.1. pattern-eight
4.4.1.2. Circles
4.4.1.3. Horizontal trajectory
4.4.2. Static
5. Functional Model
5.1. BLADE
5.1.1. Type
5.1.1.1. Rotor / Roto-Kite /
5.1.1.1.1. Horizontal Axis Wind Turbine (HAWT)
5.1.1.1.2. Blade Tip Rotor
5.1.1.1.3. Stacked
5.1.1.2. Cross wind traveling single Blade/Wing/Kite
5.1.1.2.1. Drag-power
5.1.1.2.2. Lift-power
5.1.1.3. Lift/Drag variators
5.1.1.3.1. Pitch variator
5.1.1.3.2. Flapping
5.1.1.3.3. Flutter
5.1.1.3.4. Shape variators
5.1.1.4. Unconventional
5.1.1.4.1. Magnus
5.1.1.4.2. Vertical-axis wind turbine/Savonius/etc
5.2. Energy transfer
5.2.1. Mechanical transfer
5.2.1.1. Traction
5.2.1.1.1. Loop/Belt
5.2.1.1.2. Pumping (Jojo)
5.2.1.1.3. Translation/Crosswind travel
5.2.1.1.4. Alternating/phased tugs
5.2.1.2. Torsion
5.2.1.2.1. Shaft
5.2.1.2.2. Rope/Cable/Tether
5.2.1.2.3. Strutted tethers / Open Tensegrity
5.2.1.2.4. Bow
5.2.1.3. (Transversal) mechanical wave
5.2.2. Electricity
5.2.2.1. Conductive tether
5.2.2.2. Untethered/Storage&transport
5.2.3. Gas
5.2.3.1. Compression
5.2.3.2. Depression
5.2.3.3. (Sound) Waves
5.2.3.4. Guided/redirected flow
5.2.4. Liquids
5.2.4.1. Hydraulic
5.2.5. Electromagnetic waves
5.2.5.1. Induction (Resonant inductive coupling)
5.2.5.2. Guided waves / one wire electricity transfer
5.2.5.3. Laser
5.2.5.4. Microwaves
5.2.6. Heat
5.2.6.1. Cooling
5.2.6.2. Steam/Evaporation
5.2.6.3. Storage&transport
5.2.7. Chemical
5.2.7.1. Hydrogen
5.2.8. Ions/Electrons
5.3. LIFT
5.3.1. Buoyant lift / Lighter than air
5.3.2. Aerodynamic lift
5.3.2.1. Kite
5.3.2.1.1. Kite Arch
5.3.2.1.2. single skin
5.3.2.1.3. Parafoil
5.3.2.2. Bernoulli
5.3.2.2.1. Plane
5.3.2.2.2. Gyroplane/autogyro
5.3.2.3. Magnus
5.3.3. Mix (kytoon)
5.4. Generator
5.4.1. Electromagnetic
5.4.1.1. Rotary
5.4.1.2. Linear
5.4.2. Piezoelectric
5.4.3. Thermoelectric (Seebeck)
5.4.4. Photoelectric (PV)
5.4.5. Inverse Magnetostriction
5.4.6. Non Electric Power
5.4.6.1. Water pump
5.4.6.2. Air Pump
5.4.6.3. Propulsive
5.4.6.3.1. Sea
5.4.6.3.2. Land