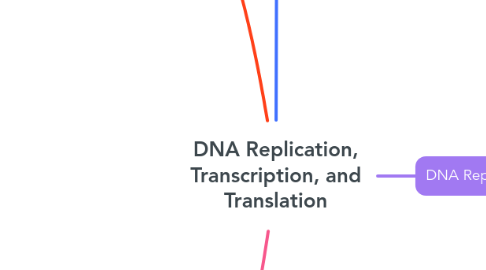
1. Translation
1.1. The process of using an mRNA template to build a protein
1.1.1. Location
1.1.1.1. It occurs on the ribosomes of the rough endoplasmic reticulum (rER) or of the cytoplasm
1.1.2. Key players
1.1.2.1. Ribosome (ribosomal RNA --> rRNA)
1.1.2.1.1. Protein Factories
1.1.2.2. Transfer RNA (tRNA)
1.1.2.2.1. It’s an RNA molecule consisting of an anticodon (complementary to the codon) and an amino acid that matches the anticodon.
1.1.2.3. Messenger RNA (mRNA)
1.1.2.3.1. The product of transcription. Every 3 nucleotides in the mRNA is known as a codon.
1.1.3. Relevance
1.1.3.1. Proteins code for traits. They are involved in everything in our body.
1.1.4. What happens here?
1.1.4.1. The mRNA attaches to the ribosome and the tRNA to the start codon in the mRNA.
1.1.4.2. The tRNA reads the mRNA codon and brings complementary anti-codons that code for amino acids to the ribosome.
1.1.4.3. Then a stop codon is reached and the mRNA detaches from the ribosome and the tRNA.
1.1.4.4. The chain of amino acids proceeds to fold into a specific shape, creating a protein.
1.1.5. What is the product of translation?
1.1.5.1. Protein: A polypeptide chain
2. Transcription
2.1. The process by of converting the genetic information from DNA to an RNA format called mRNA with the help of the RNA polymerase.
2.1.1. Location
2.1.1.1. It happens in the nucleus of eukaryotic cells.
2.1.1.2. It happens in the the cytoplasm of prokaryotic cells.
2.1.2. Relevance
2.1.2.1. Because our cells cannot make proteins directly from DNA. It’s necessary to transfer the information into an RNA intermediate called Messenger RNA (mRNA).
2.1.2.1.1. The sequence of DNA that is transcribed into RNA is called a gene
2.1.3. What happens here?
2.1.3.1. The RNA polymerase attaches to the promoter sequence in the DNA by complementary base pairing.
2.1.3.2. RNA polymerase makes a copy of the DNA strand in RNA format (mRNA) using complementary base pairs (A with U and G with C).
2.1.3.3. When the RNA polymerase reaches a termination sequence in the gene, the mRNA strand completes, and detaches from the DNA.
2.1.3.4. The product of transcription is mRNA. And it moves out of the nucleus of eukaryotic cells and into the cytoplasm to find a ribosome and start translation.
2.1.4. Why is RNA polymerase so important?
2.1.4.1. The mRNA copy is made by RNA polymerase
3. Mutations
3.1. A change in the DNA sequence that can be caused by errors in DNA replication or by damage caused by external causes.
3.1.1. Mutations can be inherited or acquired during a person's lifetime.
3.1.1.1. Only mutations that happen in sex cells are inherited.
3.1.2. Mutagens
3.1.2.1. Chemical, physical or biological elements that cause mutations, random changes, or damage to the DNA sequence.
3.1.2.1.1. The more you are exposed the more likely you are to develop a mutation.
3.1.3. Point mutations
3.1.3.1. Silent = No change in the final protein product
3.1.3.2. Missense = The mutation leads to a different amino acid than that of the original sequence
3.1.3.3. Nonsense = The mutation codes for a premature ‘STOP’ codon
3.1.4. Frameshift mutations
3.1.4.1. It’s a harmful change as the mutation shifts the way the sequence is read
3.1.4.1.1. Deletion = Results in the removal of a nucleotide from the gene.
3.1.4.1.2. Insertion = Results in the addition of a new nucleotide to the gene.
4. DNA Replication
4.1. Location
4.1.1. In eukaryotic cells, DNA replication happens inside the nucleus.
4.1.1.1. Eukaryotes organize their DNA in chromosomes which consist of DNA and histone proteins.
4.1.2. In prokaryotic cells, DNA replication happens in a region called the nucleoid
4.1.2.1. Prokaryotes organize their DNA into circular chromosomes (no histone proteins).
4.2. Models
4.2.1. Meselson and Stahl grew E.coli bacteria in an environment that contained the heavy nitrogen isotope (N-15) instead of the light-weight nitrogen isotope (N-14) in order to recall the results of how bacteria replicate when exposed to N-14.
4.2.1.1. Conservative = In this model an entirely new DNA molecule is synthesised from a DNA template
4.2.1.2. Semiconservative = In this model DNA is composed of a newly synthesized (daughter) strand and an old (parental) strand.
4.2.1.3. Dispersive = The DNA strands in this model are patchworked with both the old and the new DNA.
4.3. Relevance
4.3.1. Cells need to replace damaged DNA
4.3.2. Cells need to make a copy of DNA prior to cell division.
4.4. When does it occur?
4.4.1. S-phase of interphase
4.5. Enzymes
4.5.1. Helicase
4.5.1.1. Unwinds the DNA double helix into single strands by breaking the hydrogen bonds between nitrogenous bases
4.5.2. Primase
4.5.2.1. Adds RNA primers for DNA Polymerase to know where to start replicating a new strand
4.5.3. DNA Polymerase
4.5.3.1. Builds a new complementary and antiparallel DNA strand in a 5’ → 3’ direction.
4.5.3.1.1. This is easy on the leading strand (3’ → 5’)
4.5.3.1.2. But since the lagging strand is (5’ → 3’) and DNA polymerase can only work in a 5’ → 3’ direction, it works backwards.
4.5.4. Ligase
4.5.4.1. Seals the gaps between the old (parental) strand and the new (daughter) strand
4.6. Complementary base pairing
4.6.1. Adenine with Thymine
4.6.1.1. Double H-bond
4.6.2. Guanine with Cytosine
4.6.2.1. Triple H-bond
4.7. Structure of a DNA molecule
4.7.1. The structure of DNA is double stranded and antiparallel, which means that the strands run in an opposite direction to each other. Its shape is a double-helix and it’s made of a sugar backbone (deoxyribose), and phosphate groups that are attached to one of the nitrogenous bases.