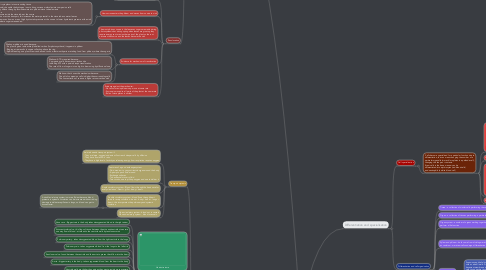
1. Differentiation and specialisation
1.1. Cell specialisation
1.1.1. -Cells become specialised for a particular function due to differentiation; this can mean changing the number of a particular organelle (more chloroplasts in a palisade cell), changing the shape or contents. -Stem cells in the bone marrow can be differentiated into erythrocytes (red blood cells) and neutrophils (a white blood cell)
1.1.1.1. Erythrocytes
1.1.1.1.1. -Lose their nucleus, mitochondria, golgi apparatus and rough ER and are full of haemoglobin. They are also biconcave. -They can transport large amounts of oxygen around the body
1.1.1.2. Neutrophils
1.1.1.2.1. -Granular cytoplasm due to large number of lysosomes -Ingest and digest invading pathogens with enzymes from lysosomes
1.1.1.3. Sperm cells
1.1.1.3.1. -Many mitochondria for the movement of undulipodium -Acrosome in the head is a lysosome that contains enzymes to penetrate the egg -Streamlined shape to aid movement -Undulipodium helps to propel the cell up the uterine tract -Gamete
1.1.1.4. Root hair cells
1.1.1.4.1. -Large surface area to absorb water and minerals from the soil
1.2. Differentiation and cell organisation
1.2.1. Tissue - a collection of similar cells performing the same function
1.2.2. Organ - a collection of tissues performing a particular function
1.2.3. Organ system - a number of organs working together to perform a life function
1.2.4. Xylem and phloem - both come from dividing meristem cells such as cambium - meristem cells undergo differentiation
1.2.4.1. Xylem - consists of vessels with parenchyma cells and fibres. Walls are reinforced with lignin and end walls break down to form a continuous tube with a wide lumen. Transports water and minerals up the plant
1.2.4.2. Phloem - Consists of sieve tubes and companion cells. Cells line up to form a long tube, with cells separated by sieve plates that are perforated to allow movement of materials up or down the tubes. Companion cells are metabolically active and are involved in the movement of sugars into the phloem
1.2.5. Epithelial tissues
1.2.5.1. Squamous epithelial tissue - flattened cells that form a thin, smooth, flat surface such as alveoli walls. Provides a short diffusion distance. Cells are held in place by a basement membrane made of collagen and glycoproteins which attached epithelial cells to connective tissue.
1.2.5.2. Ciliated epithelial tissue - contains ciliated cells which waft mucus (produced by goblet cells) up the respiratory tract. Damaged by nicotine and tar
1.2.6. Leaves
1.2.6.1. Leaves are adapted to perform photosynthesis efficiently
1.2.6.2. Waxy cuticle; a transparent upper surface that lets light through but prevents water loss
1.2.6.3. Palisade cells; contain large number of chloroplasts containing chlorophyll
1.2.6.4. Spongy mesophyll; a layer of cells with air spaces to allow circulation of gases
1.2.6.5. Lower epidermis; contains pores called stomata surrounded by two guard cells which open to allow gas exchange and close to prevent water loss. The opening of the stomata is due to water moving into guard cells making them turgid which causes a pore to form as they bulge at both ends
2. The Lungs and gas exchange
2.1. Exchange surfaces
2.1.1. -Single celled and small organisms can exchange gases/nutrients across their outer surface due to a large surface area to volume ratio -Larger organisms have a smaller ratio therefore have a greater distance between the surface and the centre so need specialised exchange surfaces -Specialised exchange surfaces are small intestine, liver, root hairs of plants and hyphae of fungi
2.1.1.1. A good exchange system: -Large surface area -Thin diffusion distance -Fresh supply of molecules on one side and removal on the other to maintain concentration gradient
2.2. Adaptations of the lung
2.2.1. -Air travels through the nose > trachea > bronchi > bronchioles > alveoli where gas exchange happens -Oxygen passes through the alveoli into capillaries whiles carbon dioxide travels other way -Alveoli have large surface area -Small diffusion distance: alveolus wall and capillary wall are one cell thick. Capillaries are close to the wall and are narrow so red blood cells are against the capillary wall. -Alveoli are moist to allow gases to diffuse, covered in surfactant to reduce cohesion between water molecules; otherwise they would collapse -Diffusion gradient is maintained as blood carries oxygen away from the lungs and brings supply of carbon dioxide. There is a high CO2 and low oxygen concentration in the red blood cells and opposite in alveolus.
2.2.1.1. Inspiration
2.2.1.1.1. 1. Diaphragm contracts and flattens 2. Intercostal muscles contract and ribs rise 3. Volume of chest cavity increases 4. Pressure in chest decreases below atmospheric pressure 5. Air moves into lungs
2.2.1.2. Expiration
2.2.1.2.1. 1. Diaphragm relaxes and is pushed up 2. Intercostal muscles relax and ribs fall 3. Volume of chest cavity decreases 4. Pressure in chest cavity increases about atmospheric pressure 5. Air moves out of lungs
2.3. Structure of the respiratory system
2.3.1. Cartilage - provides structure and support to hold airways open
2.3.2. Elastic fibres - recoil to dilate the airway after smooth muscles contract
2.3.3. Smooth muscle - contracts to constrict the airway (narrows lumen) to restrict airflow to alveoli
2.3.4. Goblet cells - Secrete mucus to trap dust/pollen/bacteria
2.3.5. Ciliated epithelium - ciliated cells waft mucus up the airway to be swallowed
2.3.6. Structure of the trachea - C shaped ring of cartilage to allow flexibility, elastic fibres, smooth muscle, and blood vessels. the epithelium layer has goblet cells and ciliated cells.
2.3.7. Structure of bronchi - same as trachea but smaller and less cartilage
2.3.8. Bronchioles - no cartilage but has smooth muscle, elastic fibres and a ciliated epithelium
2.3.9. Alveoli - Smooth muscle to contract and expand alveoli and elastic fibres to recoil to allow them to expel waste gases
2.4. Measuring lung capacity
2.4.1. Volume of air moved in and out during breathing measured by spirometer. Person breaths through a disposable mouth piece (disinfected) into a tank filled with medical grade oxygen; a lid moves up and down to produce a trace
2.4.2. Soda lime absorbs any exhaled carbon dioxide
2.4.3. Oxygen uptake calculated as the amount of carbon dioxide breathed out is the same as the volume of oxygen breathed in - so the total amount of air in the chamber will decrease depending on oxygen uptake
2.4.4. Tidal volume - Volume breathed in and out at rest
2.4.5. Vital capacity - largest amount of air breathed in and out in one breath
2.4.6. Residual volume - volume of air that stays in lungs
2.4.7. Dead space - air in bronchioles, bronchi and trachea
2.4.8. Inspiratory reserve volume - extra air breathed in above normal tidal volume
2.4.9. Expiratory reserve volume - extra air breathed out above normal tidal volume
3. The heart and circulatory system
3.1. Transport systems
3.1.1. Animals need a transport system if: - They are large; oxygen/nutrients will not reach deeper cells by diffusion - They have a small SA:V ratio -They have a high level of activity as releasing energy from respiration requires oxygen
3.1.2. Features of a good exchange system: -Fluid medium to carry nutrients/oxygen around the body -A pump to push fluid around -Exchange surfaces - Tubes/vessels to carry fluid -Two circuits; one to pick up oxygen and one to deliver it
3.1.3. Single circulatory system - Blood flows through the heart once for every circulation - Heart > gills > body > heart
3.1.4. Double circulatory system - blood flows through heart twice for every circulation - heart > body > heart > lungs > heart - this incorporates the pulmonary and systemic cirulation
3.1.4.1. A double cirulatory system is more efficient because blood pressure in systemic circulation can be increased without ricking damage to delicate capillaries in lungs, so blood can get to tissues faster.
3.1.5. Open circulatory system - blood not in vessels Closed circulatory system - blood in vessels.
3.2. Heart structure
3.2.1. Vena cava - Biggest vein in the body takes deoxygenated blood to the right atrium
3.2.2. Atrioventricular valve - thin flaps of tissue between the atria and ventricles to ensure one way flow of blood - attached to the ventricle walls by tendinous cords
3.2.3. Pulmonary artery - takes deoxygenated blood from the right ventricle to the lungs
3.2.4. Pulmonary vein - takes oxygenated blood from the lungs to the left atria
3.2.5. Semi lunar valve - found between the ventricle and the aorta to prevent back flow into the heart
3.2.6. Aorta - biggest artery in the body - takes oxygenated blood from the heart to the body
3.2.7. Ventricle walls are thicker than atria as they must provide more pressure
3.2.8. The walls of the right ventricle are less thick than the left as it doesn't need to provide as much pressure since the distance between the lungs and heart is less and high pressure would damage thin capillaries in the lungs.
3.3. The Cardiac Cycle
3.3.1. 1. Atrial systole - atria contracts -The pressure in atria is higher than that in the ventricles, causing the AV valve to open and blood to flow in the ventricles 2. Ventricular systole - ventricles contract -Pressure in ventricles is higher than atria so AV close. -Pressure in ventricles is higher than in arteries so semi lunar valve opens. -Heart muscle starts to relax and elastic tissues in ventricle walls recoils -Pressure in the ventricle falls below that in the arteries so semilunar valve closes 3. Diastole - atria and ventricles relax whilst blood flows into the atria
3.3.1.1. Control of cardiac cycle: -Cardiac muscle is myogenic; initiates its own contraction -Sinoatrial node initiates a wave of excitation; known as pacemaker -Wave of excitation spreads over atria and atria contracts - The atrioventricular node (on the septum between ventricles) delays the excitation to allow atria to finish contracting - The wave if excitation runs down the Purkyne tissue to to the apex of the heart before spreading across the ventricle walls from the base upwards - pushing blood into arteries.
3.3.2. ECGs measure electrical activity in the heart: -P peak indicates excitation of atria, QRS peak indicates ventricular excitation, T peak shows diastole
3.3.2.1. Elevation of ST indicates heart attack
3.3.2.2. Small/unclear P wave indicates atrial fibrillation - inefficient pumping
3.3.2.3. Deep S wave indicates abnormal increased muscle thickness
3.4. Blood Vessels
3.4.1. Arteries: - Small lumen for high pressure -Thick wall containing collagen to withstand high pressure -Elastic tissue to stretch and recoil to maintain high pressure -Smooth muscle to constrict the artery and limit blood flow -Folded endothelium that can unfold when artery stretches
3.4.2. Veins: - Large lumen to ease blood flow - Thinner layers of collagen/smooth muscle/elastic tissue as they do not need to stretch and recoil -Valves to prevent backflow of blood
3.4.3. Capillaries: - Walls are one cell thick and flattened and very narrow lumen to squeeze red blood cells to help them give up oxygen by reducing diffusion distance
3.5. Blood, tissue fluid and Lymph
3.5.1. -Blood is held in the heart/blood vessels, tissue fluid bathes tissues and lymph is held in the lymphatic system -When arteries reach tissues they branch into arterioles and then capillaries -Hydrostatic pressures pushes fluid out the blood and into the tissue fluid -Effect of water potential draws fluid into the blood from the tissue fluid; the water potential in the capillary is lower than in the tissue fluid due to dissolved solutes -At the arteriole end of the capillary, hydrostatic pressure is higher than the effect of water potential so fluid moves out of the capillary into the tissue fluid by diffusion taking useful products (oxygen) with it -At the venule end of the capillary, hydrostatic pressure is lower than the effect of water potential so fluid moves back into the capillary by osmosis, bringing waste products (carbon dioxide) with it -Some tissue fluid drains into the lymphatic system which rejoins the blood in the chest cavity; lymph contains lymphocytes produced in lymph nodes which engulf and destroy pathogens
3.6. Carriage of gases
3.6.1. Oxygen
3.6.1.1. -Transported by erythrocytes containing haemoglobin to form oxyhaemoglobin -Haemoglobin has 4 subunits with haem groups containing an iron ion which holds oxygen molecule; it has an affinity for oxygen so each haemoglobin molecule can hold 4 oxygen molecules -Haemoglobin takes oxygen out of solution to maintain a concentration gradient so more oxygen can enter -Dissociation is the release of oxygen into cells -Partial pressure is a measure of amount of oxygen present -As partial pressure of oxygen in the surroundings increase, so does the haemoglobin percentage saturation; it forms an S- shaped oxygen dissociation curve because: -It is hard for the first oxygen molecule to diffuse into the cell as the group that attracts it is in the centre of the molecule -The first oxygen binding causes a conformational change in the shape, making it easier for the second and third to bind -It is harder for the last oxygen to diffuse in and associate with the last haem group; it is difficult to achieve 100% saturation -Oxygen partial pressure in the lungs is high to allow almost 100% saturation but sufficiently low in the tissue to cause dissociation of oxygen for use in respiration -Foetal haemoglobin has a higher affinity for oxygen so that it can pick up oxygen from an environment that makes adult haemoglobin dissociate -Adults have different haemoglobin to allow dissociation in tissues as foetal haemogloblin would not dissociate -Foetal heamoglobin curve is to the left of the adult curve
3.6.2. Carbon dioxide
3.6.2.1. -Carbon dioxide is transported in the blood in three ways; 5% dissolved in plasma, 10% combined with haemoglobin to form carbaminohaemoglobin and 85% as hydrogencarbonate ions -At respiring tissues, carbon dioxide diffuses into the blood - It combines with water in red blood cells to form carbonic acid -This dissociates to form hydrogen ions and hydrogencarbonate ions -Hydrogencarbonate ions diffuse out into the plasma -Chloride ions move into the red blood cell to maintain the charge - this is chloride shift -Hydrogen ions make the cell acidic; haemoglobin takes up the hydrogen ions to form haemoglobinic acid to maintain the pH- it acts as a buffer -The hydrogen ions compete with the oxygen for space on the haemoglobin molecule; so more carbon dioxide leads to more dissociation of oxygen -Where tissues are respiring more, more carbon dioxide is released so more hydrogen ions are produced and more oxyhaemoglobin releases more oxygen; this is the Bohr effect; the oxygen dissociation curve moves down and to the right
4. Transport in plants
4.1. Distribution of xylem and phloem
4.1.1. -Multicellular plants need a transport system because cells inside the plant would not receive enough water and nutrients by diffusion alone -Water travels up from the roots in xylem tissue -Sugar travels up and down in the phloem tissue -Xylem and phloem are found together in vascular bundles -Meristem cells are pluripotent that can differentiate into xylem or phloem; they are found in the cambium in the stem and in the pericycle in the root
4.2. Structure and adaptation of xylem
4.2.1. -Xylem vessel elements are long cells with thick walls impregnated with lignin for waterproofing -The cells are dead and form a long column with no contents or end walls; this is the xylem vessel -Lignin in the walls forms patterns allowing the vessel to be flexible -Pores between the lignin are called pits which allow water to travel into adjacent vessels or pass into living parts of the plant -Tubes are narrow so the column of water does not break; this makes capillary action effective -Water flow is not impeded as there are no end walls, no contents and a lignin wall to prevent collapse
4.3. Movement of water
4.3.1. Movement between cells: -Water moves from a high water potential to and area of low water potential -Cytoplasm in plants has a low water potential due to dissolved solutes -A plant cell placed in pure water will absorb water by osmosis and become turgid -Apoplast pathway - Water moves through spaces in cell walls and between cells; dissolved mineral ions can be carried as water does not cross a plasma membrane -Symplast pathway - water travels through plasma membranes and cytoplasm and moves between cells through plasmodesmata -Vacluolar pathway - water is able to pass through vacuoles as well as cytoplasm
4.3.2. Movement across the root: -Root hair cells increase surface area of the root and absorb minerals from the soil by active transport using ATP -The minerals reduce water potential of the cell so water is taken up by osmosis down a water potential gradient -The endodermis (layer of cells surrounding the xylem) actively transports minerals from the cortex (cells between the root hair and endodermis) into the xylem so water flows by osmosis -Casparian strip - a waterproof strip in the walls of endodermic cells which blocks the apoplast pathway and forces water into the symplast pathway; this ensures that water and nitrate ions have to pass through a plasma membreane so that the nitrate ions can be actively transported from the cytoplasm of cortex cells into the xylem to reduce water potential, causing water to follow.
4.3.3. Movement up the stem
4.3.3.1. Root pressure: -Endodermal cells actively transporting minerals into the xylem drives water in via osmosis -The water being forced into the xylem pushes water up the stem only by a few metres
4.3.3.2. Transpiration pull: -Water molecules are attached to each other by cohesion so form a continuous coloumn -As water leaves the leaf the whole column is pulled up creating a transpiration stream -The pull from above creates tension; hence the term cohesion-tension theory -If a water column is in one xylem vessel it can be maintained through another vessel via the pits
4.3.3.3. Capillary action: -Water molecules adhere to the sides of the xylem vessel -As the vessels are narrow, the forces of attraction can pull the water up the sides of the vessel
4.3.3.4. -Water leaves through the leaf through stomata which are opened and closed by guard cells -The water evaporates from the cells lining the cavity immediately below the guard cells, lowering the water potential and causing more water to enter them via osmosis from neighbouring cells
4.4. Transpiration and xerophytes
4.4.1. - Transpiration is the loss of water by evaporation from the aerial parts of the plant - This is unavoidable as water leaves through open stomata which must open for photosynthesis gas exchange.
4.4.2. The process of transpiration: -Water enters the leaves in xylem and passes to mesophyll cells by osmosis -Water evaporates form the surface of cells to form vapour -Water vapour collects in air spaces until the water potential inside the leaf is higher than the outside -Water vapour diffuses out of the leaf through open stomata
4.4.3. Factors affecting the rate of water loss
4.4.3.1. Number of leaves - more leaves means larger surface are so water vapour is lost faster
4.4.3.2. Number/size of stomata - more/bigger stomata leads to more water loss
4.4.3.3. Position of stomata - stomata on the underside of leaves reduce the rate of water loss
4.4.3.4. Waxy cuticle - reduces evaporation
4.4.3.5. Light- causes stomata to open to allow gas exchange for photosynthesis so water can be lost
4.4.3.6. Temperature - higher temperature increases rate of evaporation and diffusion through stomata as water molecules have more kinetic energy but decreases relative water vapour potential in the air allowing more rapid diffusion
4.4.3.7. Relative humidity - higher humidity in the air decreases water loss as water vapour potential gradient is smaller
4.4.3.8. Wind - wind carries water vapour away from the plant so increases the water vapour potential gradient
4.4.3.9. Water availability - little water in the soil means that plants cannot replace water lost so this is reduced
4.4.4. Adaptations of xerophytes
4.4.4.1. Small needle-shaped leaves to reduce surface area so less water is lost
4.4.4.2. Densely packed spongy mesophyll - reduces surface area exposed to air inside the leaves so less evaporation
4.4.4.3. Thick waxy cuticle - reduces evaporation
4.4.4.4. Closing stomata when water availability is low
4.4.4.5. Hairs on surface - trap air close to the surface which becomes saturated with moisture, thus reducing water potential gradient
4.4.4.6. Pits containing stomata - trap air that becomes saturated with moisture
4.4.4.7. Rolling leaves - lower epidermis is not exposed to the atmosphere and air can be trapped
4.4.4.8. High salt concentration inside cells - decreases water potential inside cell to reduce the water potential gradient between the inside and outside of the leaf
4.4.4.9. Marram grass is an example of a xerophyte with characteristic adaptations
4.5. Structure and adaptations of phloem
4.5.1. -Phloem consists of sieve tube elements and companion cells -Sieve tube elements contain little cytoplasm and no nucleus; lined up to form a tube -Perforated cross-walls (sieve plates) allow sap to flow through -Companion cells have a large nucleus and dense cytoplasm, as well as lots of mitochondria to carry out respiration and release energy in the form of ATP -The ATP is a source of energy for loading sucrose into sieve tubes -Companion cells are linked to sieve tube elements through plasmodesmata (gaps in the cell walls)
4.6. Translocation
4.6.1. - Translocation is the movement of assimilates (sugars/other other chemicals made by plant cells) in the phloem tissue -A source releases sucrose into the phloem -A sink removes sucrose from the phloem
4.6.2. How sucrose enters the phloem and moves from source to sink
4.6.2.1. - Companion cells use ATP to actively transport hydrogen ions out of their cytoplasm into surrounding tissue -Hydrogen ions diffuse back into the cells through cotransportor proteins which enable the hydrogen ions to bring sucrose molecules into companion cells -Concentration of sucrose molecules in companion cells build up, so they diffuse through plasmodesmata into phloem sieve tube elements -Sucrose entering the phloem reduces the water potential in the sieve tube -Water moves into the sieve tube by osmosis, increasing hydrostatic pressure inside the sieve tube at the source - At the sink, sucrose moves out of the cell to respiring tissues by diffusion or active transport; this increases the water potential in the sieve tube so water leaves -The hydrostatic pressure at the sink decreases, so water carrying sucrose moves from an area of high hydrostatic pressure at the source to lower hydrostatic pressure at the sink -This is called mass flow and can occur in either direction depending on where sugars are needed
4.6.3. The most obvious source is the leaves as sugars are made during photosynthesis but during spring when leaves are growing they require energy so stores in other parts of the plant so the root becomes the source and the leaves become the sink
4.6.4. Evidence for mechanism of translocation
4.6.4.1. We know phloem is used because: -If a plant is given radioactively labelled carbon (for photosynthesis) it appears in phloem -Ringing a tree results in sugars collecting above the ring -Aphids feeding on a plant stem can be used to show that mouthparts are taking food from phloem; where the sugar is
4.6.4.2. We know ATP is required because: -Companion cells have many mitochondria -inhibiting ATP with a poision stops translocation -The rate of flow of sugars is too high to be moving by diffusion alone
4.6.4.3. We know that it uses this mechanism because: -The pH of companion cells is higher than surrounding cells -The concentration of sucrose is higher at source than sink
4.6.5. Evidence against the mechanism: - Not all solutes in phloem sap move at same rate - Sucrose is moved to all parts of the plant at the same rate - Role of sieve plates is unclear