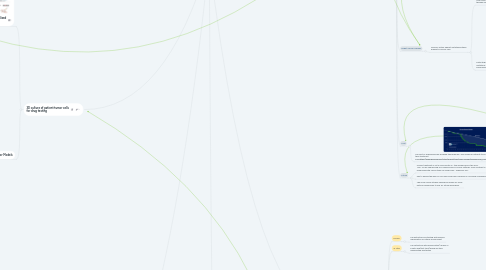
1. Use of animal models (mice, zebrafish, avatar models) for implanting patient tumor cells to test anti-cancer drugs
1.1. Xenograft- a tissue graft or organ transplant from a donor of a different species from the recipient.
1.2. The doctor collects the tumor cells from the patients. Then the cells are analyzed and injected into the animal of choice. The tumor is then watched and after some time (couple weeks) the tumor cells are then collected from the animal and analyzed. From there drugs are make and tried on the to see if they work and what kind of toxicities they cause. If the drug works and is safe then it injected into the patient. This makes it personalized medicine.
1.2.1. Mice
1.2.1.1. Patient-derived xenograft (PDX) models have been extensively used in studies of various solid and hematologic malignancies, such as breast cancer, colorectal cancer, pancreatic cancer, chronic lymphocytic leukemia, and large B cell lymphoma. PDX models are used for the assessment of human tumor biology, identification of therapeutic targets, and are an important model for preclinical testing of new drugs for various cancers. PDX models are established by the implantation of cancer cells or tissues from patient primary tumors into immunodeficient mice.
1.2.1.1.1. To implant patient-derived tumors into immunodeficient mice, small fragments of tumors, cell suspensions derived from blood or from the digestion of tumors into single-cell suspensions are used. The implantation can be performed heterotopically or orthotopically.
1.2.2. Zebrafish
1.2.2.1. Adult zebrafish xenografts are similar to mouse xenografts but have significant experimental advantages. The zebrafish xenograft assay is ideal for personalized medicine. Imaging of the small, transparent fry is unparalleled among vertebrate organisms. Zebrafish develops cancer phenotypes similar to human cancer in different tissues and organs.
1.2.2.1.1. https://cancerres.aacrjournals.org/content/canres/78/21/6048/F1.large.jpg
1.2.3. Avatar Models
1.2.3.1. A subset of PDXs, referred to as Avatars, establish tumors in an orthotopic and treatment naïve fashion that may represent the most clinical relevant model of individual human cancers.
1.2.3.1.1. Avatar models allow evaluation of drug response, safety, and efficacy in cancer patients with good concordance to the corresponding patient original tumors. They have the potential to provide significant improvement in clinical outcomes, and for defining optimal patients for clinical trials. Despite limitations to their use including further investigation in regards to microenvironment mimicry and metastatic case applicability, we feel they provide a promising approach to facilitate future research in OC.
1.2.3.1.2. Patient Avatar models can be important instruments in foreseeing which patients may benefit from the utilization of platinum-based therapies, and highlighting those for whom platinum may have limited value, who would require different treatments in the short-term.
1.2.3.1.3. “Avatars” have allowed us to transplant tumor tissue into multiple mice then harvest them at different time points; allowing a more complete understanding of these mechanisms
2. 3D culture of patient tumor cells for drug testing
2.1. 3D Tumor Models in Personalized Medicine
2.1.1. (A) Starting materials for tumor spheroids and organoids cultivations
2.1.1.1. Cancer stem cells
2.1.1.2. Cancer cells
2.1.1.3. Cancer tissue
2.1.2. (B) Structure of Tumor Spheroid
2.1.2.1. Inner Necrotic zone
2.1.2.2. Senescent zone
2.1.2.3. Outer Proliferation zone
2.1.3. (C) Application of tumor spheroids and organoids
2.1.3.1. Drug screening, discovery, and chemosensitivity testing
2.1.3.2. Assessment of various multi-"omics" profiles (e.g., transcriptomics, genomics, etc.), comparison with a native tumor and therapeutic target search
2.1.3.3. Modeling tumor microenvironment and angiogensis
2.1.3.4. Xenograph models (studying the patterns of tumor growth and in vivo drug valdiation)
2.1.3.5. Biobank that can be used to screen drugs and facilitate development of therapeutic agents
2.2. Types of 3D Tumor Models
2.2.1. Spheroids
2.2.1.1. Self-organizing cultures of tumor cells with a rounded morphology and predominant intercellular interactions that can proliferate and grow as free-floating structures
2.2.1.1.1. 3 Frequently Used Spherical Models
2.2.1.2. Spheroid Advantages
2.2.1.2.1. Possibility of creation of co-culture, culturing cells without special equipment, and cultures without expensive cultivation methods
2.2.1.2.2. Gas, nutrients, and pH gradients are present
2.2.1.3. Spheroid Disadvantages
2.2.1.3.1. Difficulty of forming homogenous spheroids
2.2.1.3.2. Fragile spheroid structure
2.2.1.3.3. Gradient structure complicates drug testing
2.2.2. Organoids
2.2.2.1. 3D culture of normal cells and tissues; aggregates of different cell types, often used in combination with a biocompatible 3D scaffold
2.2.2.1.1. Capable of self-renewal, self-organization, and manifestation of functionality of organ that it imitates
2.2.2.1.2. Maintain cellular composition and structure similar to tissue from which they originate
2.2.2.1.3. Intratumoral heterogeneity: genetically and phenotypically more similar to native tumor
2.2.2.2. Organoids Advantages
2.2.2.2.1. Possibility of creation of co-culture, reproduction of intercellular interactions and cell-ECM interactions, and primary tumor cells long-term cultivation
2.2.2.2.2. Stable at passaging
2.2.2.3. Organoids Disadvantages
2.2.2.3.1. Expensive method
2.2.2.3.2. Gradient of gases, nutrients, and pH not always reproducible
2.2.2.3.3. Therapeutic responses may depend on matrix
3. Companies or institutions doing ex vivo culture of patient cancer cells for drug matching
3.1. Ex vivo drug screening refers to the out-of-body assessment of drug efficacy in patient derived vital tumor cells. The purpose of these methods is to enable functional testing of patient specific efficacy of anti-cancer therapeutics and personalized treatment strategies. Such approaches could prove powerful especially in context of rare cancers for which demonstration of novel therapies is difficult due to the low numbers of patients.
3.1.1. KIYATEC
3.1.1.1. 2020 will highlight findings of the company’s ability to detect dose-dependent response to checkpoint blockade and corresponding correlation with immune cell activation in high-throughput ex vivo 3D tumor spheroid models.
3.1.1.1.1. KIYATEC believes these recent advances may represent key building blocks toward the eventual development and validation of clinical assays capable of accurate pre-treatment, patient-specific prediction of response to immuno-oncology drugs.
3.1.2. Abeona Therapeutics
3.1.2.1. Clinical programs include EB-101, its autologous, gene-corrected cell therapy for recessive dystrophic epidermolysis bullosa. as well as ABO-102 and ABO-101, AAV9-based gene therapies for Sanfilippo syndrome types A and B (MPS IIIA and MPS IIIB), respectively.
3.1.2.2. As well as ABO-102 and ABO-101, AAV9-based gene therapies for Sanfilippo syndrome types A and B (MPS IIIA and MPS IIIB), respectively.
3.1.3. Asklepios BioPharmaceutical
3.1.3.1. The company’s gene therapy platform includes Pro10, a proprietary cell line manufacturing process, and an extensive AAV capsid and promoter library.
3.1.3.2. The company holds more than 500 patents in areas such as AAV production and chimeric and self-complementary capsids.
3.1.4. Autolus Therapeutics
3.1.4.1. Autolus is developing next generation, programmed T cell therapies for treating cancer. Using its proprietary T cell programming technologies, the company is engineering precisely targeted, controlled, and highly active T cell therapies that are designed to better recognize cancer cells, break down their defense mechanisms and eliminate these cells.
3.1.5. Elevate Bio
3.1.5.1. ElevateBio launched HighPassBio for advancing novel targeted T cell immunotherapies initially developed at the Fred Hutchinson Cancer Research Center. AlloVir, based on technology from the Baylor College of Medicine’s Center for Cell and Gene Therapy, also is developing T cell immunotherapies.
3.1.5.1.1. Among its products, HighPassBio is developing HA-1 TCR T cell receptor T cell therapy targeting HA-1 expressing tumors designed to manage post-hematopoietic stem cell transplant leukemic relapse. AlloVir is developing Viralym-M (ALVR105), targeting BK Virus, Cytomegalovirus, Adenovirus, Epstein-Barr and Human Herpes virus.
3.1.6. Sana Biotechnology
3.1.6.1. Seattle, WA-based Sana Biotechnology is engineering cells as patient therapeutics. The company’s goal is to repair and control genes and replace missing or damaged cells in patients. The company employs more than 200 scientists, engineers, clinicians and biotechnologists.
3.1.6.1.1. In June, Sana announced the funding of all tranches of its initial financing, raising more than $700 million. The company launched in January 2019. Its efforts include making cells at scale ex-vivo to replace any damaged or missing cells in the body. Sana also is working on in-vivo delivery to specific cell types of any payload – including DNA, RNA, and proteins to reprogram cells.
3.2. l
3.2.1. Optimal patient stratification is of utmost importance in the era of personalized medicine. Individual treatment responses by functional ex vivo assays requires model systems derived from viable tumor samples.
3.2.1.1. KIYATEC
3.2.1.1.1. 2020 will highlight findings of the company’s ability to detect dose-dependent response to checkpoint blockade and corresponding correlation with immune cell activation in high-throughput ex vivo 3D tumor spheroid models.
3.2.1.2. Abeona Therapeutics
3.2.1.2.1. Clinical programs include EB-101, its autologous, gene-corrected cell therapy for recessive dystrophic epidermolysis bullosa. as well as ABO-102 and ABO-101, AAV9-based gene therapies for Sanfilippo syndrome types A and B (MPS IIIA and MPS IIIB), respectively.
3.2.1.2.2. As well as ABO-102 and ABO-101, AAV9-based gene therapies for Sanfilippo syndrome types A and B (MPS IIIA and MPS IIIB), respectively.
3.2.1.3. Asklepios BioPharmaceutical
3.2.1.3.1. The company’s gene therapy platform includes Pro10, a proprietary cell line manufacturing process, and an extensive AAV capsid and promoter library.
3.2.1.3.2. The company holds more than 500 patents in areas such as AAV production and chimeric and self-complementary capsids.
3.2.1.4. Autolus Therapeutics
3.2.1.4.1. Autolus is developing next generation, programmed T cell therapies for treating cancer. Using its proprietary T cell programming technologies, the company is engineering precisely targeted, controlled, and highly active T cell therapies that are designed to better recognize cancer cells, break down their defense mechanisms and eliminate these cells.
3.2.1.5. Elevate Bio
3.2.1.5.1. ElevateBio launched HighPassBio for advancing novel targeted T cell immunotherapies initially developed at the Fred Hutchinson Cancer Research Center. AlloVir, based on technology from the Baylor College of Medicine’s Center for Cell and Gene Therapy, also is developing T cell immunotherapies.
3.2.1.6. Sana Biotechnology
3.2.1.6.1. Seattle, WA-based Sana Biotechnology is engineering cells as patient therapeutics. The company’s goal is to repair and control genes and replace missing or damaged cells in patients. The company employs more than 200 scientists, engineers, clinicians and biotechnologists.
4. Commonly associated with breast & ovarian cancer
5. DNA and RNA Sequencing of cancer cells from tumors
5.1. What Genes are causing your cancer?
5.1.1. What is DNA & RNA Sequencing?
5.1.1.1. RNA Sequencing (RNA-Seq)
5.1.1.1.1. What is RNA Sequencing?
5.1.1.1.2. What do the results tell us?
5.1.1.1.3. Technique: Next Generation Sequencing (NGS)
5.1.1.2. DNA Sequencing
5.1.1.2.1. What is DNA sequencing?
5.1.1.2.2. What do the results tell us?
5.1.1.2.3. Technique: Next Generation Sequencing (NGS)
5.1.2. Target Cancer Therapy
5.1.2.1. Specific action against mutated proteins present in cancer cells.
5.1.2.1.1. How NGS is used for targeted therapy:Targeted therapy according to next generation sequencing-based panel sequencing
5.1.2.1.2. Detecting gene presence and mutations allows for Personalized Medicine
5.1.3. Cost
5.1.3.1. The cost of sequencing has dropped tremendously. This allows for patients to access sequencing to improve their treatment plan.https://www.genome.gov/sites/default/files/inline-images/Sequencing_Cost_per_Genome_May2020.jpg
5.1.3.1.1. 2003: It took more than 13 years and cost approximately €2.3B ($2.7B) to sequence an entire genome
5.1.3.1.2. 2020: Clinical whole-exome sequencing tests cost $1,000-$5,000; Whole-genome sequencing (WGS) cost about $10,000. Results are available within days.
5.1.4. Future
5.1.4.1. Cancer treatment is not a one size fits all - the answers are in the DNA: Aim: To use Sequencing on a regular basis in clinical settings. Once a patient is diagnosed with cancer they can simply say “ Sequence me”
5.1.4.2. Key to paving the way for successful precision medicine in oncology management
5.1.4.3. The more clinical studies being done allows for more data for researchers to use for future discoveries
6. In vitro drug testing of anti-cancer drugs based on genetic mutations present on the tumor
6.1. Ex vivo
6.1.1. Cell extraction and testing with minimal manipulation of natural environment
6.2. In Vitro
6.2.1. Cell extraction with amplification (usually in a petri dish/test tube) which are then manipulated and tested
6.3. Cancer treamtnent with non-mutation
6.3.1. Herbal Therapy
6.3.1.1. Chemotherapy
6.3.1.1.1. Single Anticancer drug agents:Anticancer Drug Discovery — From Serendipity to Rational Design | IntechOpen
6.4. Cancer Treatment with mutation: Combination therapy in combating cancer
6.4.1. Herbal Therapy
6.4.1.1. Chemotherapy
6.4.1.1.1. Single Anticancer drug agents
7. Techniques to Isolate Primary Tumor Cells
7.1. 2D Monolayer Culture
7.1.1. Pros: Easy to culture from single cell suspension of tumor.
7.1.2. Cons: Contamination with non-tumorigenic cells, differentiation and genetic drift. Variability in drug responses. Less chances to retain original phenotypes.
7.2. Explant cell culture
7.2.1. Pros:Ability to remove explants with fibroblasts growth.
7.2.2. Cons:Not good for soft tissues (melanoma). Need serial subculuring to enrich tumor cells.
7.3. Precision cut culture
7.3.1. Pros:Stringent thickness might facilitate to grow tumor cells. Simple method to culture explants.
7.3.2. ConsThickness of tissue slices has to be accurate. Tissue architecture needs to be considered.:
7.4. 3D Culture
7.4.1. Pros:Optimal simulation of in vivo condition with minimal genetic drift in long term culture. Tumor cells are able to maintain 3D architecture.
7.4.2. Cons: Biomimetic scaffolds are expensive. Need expertise to generate cyto- architecture.
7.5. Partial Enzymatic Degradation of Stromal Cells
7.5.1. Pros:Easy to remove stromal and fibroblast cells. Success rate to derive from tumor is high.
7.5.2. ConsThis technique needs to be applied for various types of tumors to validate its success rate.:
7.6. Sandwich Culture
7.6.1. Pros:Suitable for isola tion of hypoxic tumor cells. Maintain in vivo tissue specific function in culture condition.
7.6.2. Cons:Isolating only a subset of tumor cells. Metabolic profile of tumor cells might change due to the glass slide barrier
7.7. Cancer Stem Cell Isolation
7.7.1. Pros:Selectively enrich clonal tumor stem cells using surface markers. Culture is highly enriched with tumor stem/initiating cells.
7.7.2. Cons:Difficult to isolate heterogeneous cancer cells. Possibility to isolate normal stem cells.
7.8. Chemical Reprogramming
7.8.1. Pros:No genetic alternation after treatment with Rock inhibitor Y-27632 for several passages. Rapid proliferation of cells. Treatment can be applied to all types of tissues.
7.8.2. Cons: It is not clear whether heterogeneous population can proliferate. The success rate of deriving cells from primary tumors is unknown.: