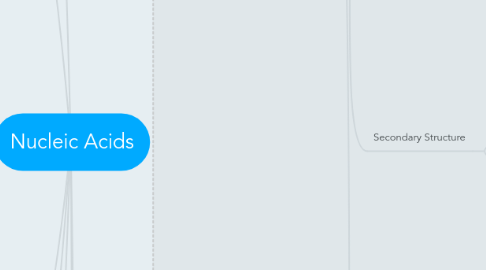
1. linear polymers of nucleotides (polynucleotides)
1.1. linked 3
1.1.1. 5
1.2. Polymers of ribonucleotides
1.2.1. RNA
1.3. polymers of deoxyribonucleic acid
1.3.1. DNA
1.4. The convention in all notations of nucleic acid structure is to read the polynucleotide chain from the 5'
1.4.1. Occasionally, a lowercase “p” is written between each successive base to indicate the phosphodiester bridge, as in GpApCpGpUpA.
2. DNA
2.1. Fundamental Structure of DNA Is a Double Helix
2.1.1. The strands run in opposite directions; that is, antiparallel.
2.1.2. held together through interchain hydrogen bonds
2.2. Chargaff’s rules: [A]
2.3. Watson and Crick’s Postulate of the DNA Double Helix
2.3.1. complementary double helix
2.3.1.1. A
2.3.1.1.1. The sequence of bases in one strand has a complementary relationship to the sequence of bases in the other strand.
2.3.1.1.2. That is, the information contained in the sequence of one strand is con- served in the sequence of the other.
2.4. DNA contains two kinds of information:
2.4.1. 1. The base sequences of genes that encode the amino acid sequences of proteins and the nucleotide sequences of functional RNA molecules such as rRNA and tRNA (see following discussion)
2.4.2. 2. The gene regulatory networks that control the expression of protein-encoding (and functional RNA-encoding) genes (see Chapter 29)
2.5. in the Form of Enormously Long, Threadlike Molecules
2.5.1. ize can be represented in terms of the numbers of paired nucleotides (or base pairs)
2.5.2. Because of their long, threadlike na- ture, DNA molecules are easily sheared into shorter fragments during isolation proce- dures,
2.6. DNA in Cells Occurs in the Form of Chromosomes
2.6.1. single chromosome of prokaryotic cells (Figure 10.19) is typi- cally a circular DNA molecule
2.6.2. DNA molecules of eukaryotic cells, each of which defines a chromosome, are linear and richly adorned with proteins
2.6.2.1. HISTONES: arginine- and lysine-rich basic proteins
2.6.2.1.1. interact ionically with the anionic phosphate groups in the DNA backbone to form nucleosomes
3. Nucleosides
3.1. nitrogenous base + a sugar pentose
3.1.1. Ribonucleosides contain the pentose D-ribose
3.1.1.1. common ribonucleosides
3.1.2. deoxyribonucleosides contain 2-deoxy-D-ribose
3.1.2.1. H instead of OH
3.2. no phosphate lang ganern
3.3. http://www.mikeblaber.org/oldwine/BCH4053/Lecture18/nucleoside01.jpg
3.4. Gly- cosidic bonds in nucleosides (and nucleotides, see following discussion) are always of the
3.5. Nucleosides are more water soluble than the free bases, because of the hydrophilicity of the pentose
4. DNA overview
4.1. information to make all the functional mac- romolecules of the cell (even DNA itself) is preserved in DNA
4.1.1. accessed through transcription of the information into RNA copies
4.2. found principally in two copies in the diploid chromosomes of the nucleus.
4.2.1. also found in mitochondria and in chloroplasts, where it encodes some of the proteins and RNAs
4.3. Two fundamental chemical differences distinguish DNA from RNA:
4.3.1. DNA contains 2-deoxyribose instead of ribose
4.3.1.1. greater resistance of DNA to alkaline hydrolysis
4.3.1.1.1. RNA Is Susceptible to Hydrolysis by Base, but DNA Is Not
4.3.2. DNA contains thymine instead of uracil.
4.3.2.1. Deamination of cytosine forms uracil.
4.3.2.1.1. mutation
4.3.3. because of these differences, DNA is chemically more stable than RNA.
5. RNA overview
5.1. central fxn: information transfer from DNA to protein
5.1.1. types
5.1.1.1. messenger RNA, ribosomal RNA, transfer RNA, and small nuclear RNA. Another type, small RNAs
6. RNA STRUCTURE
6.1. RNA molecule has a much greater number of conformational possibilities.
6.2. Although single-stranded
6.2.1. rich in double-stranded regions that form when complementary sequences within the chain come together
6.2.1.1. join via intrastrand base pairing
6.2.1.1.1. create hairpin stem-loop structures
6.2.1.1.2. cannot form B-DNA-type double helices because the RNA 2
6.3. tRNA 2ndary Structure
6.3.1. Transfer RNA Adopts Higher-Order Structure Through Intrastrand Base Pairing
6.3.1.1. a majority of the bases are hydrogen bonded to one another
6.3.1.1.1. Hairpin turns bring complementary stretches of bases in the chain into contact so that double helical regions form
6.4. trna tertiary structure
6.4.1. Tertiary structure in tRNA arises from base-pairing interac- tions between bases in the D loop with bases in the variable and T
6.5. Ribosomal RNA Also Adopts Higher-Order Structure Through Intrastrand Base Pairing
6.5.1. large degree of intrastrand sequence complementarity is found in all ribosomal RNA strands
6.5.1.1. ribosomal RNA species (regardless of source) serve common roles in protein synthesis, it may be anticipated that they share structural features.
6.5.1.1.1. All ribosomes are similar, and all function in a similar manner.
6.5.2. rRNA Tertiary Structure
6.5.3. Aptamers
6.5.3.1. synthetic oligonucleotides, usually RNA, which fold into very specific three-dimensional structures that selectively bind ligands with high affinity
6.5.4. Riboswitches
6.5.4.1. a naturally occurring class of aptamers, are conserved regions of mRNAs that reversibly bind specific metabolites and coenzymes and usually act as regulators of gene expression
7. Nucleotides
7.1. heterocyclic nitrogenous base
7.1.1. a five- carbon sugar (pentose)
7.1.1.1. phosphate
7.1.1.2. DNA: 2-deoxyribose
7.1.2. ribose RNA
7.1.3. 2-deoxyribose DNA
7.2. derivatives of either pyrimidine or purine.
7.2.1. pyrimidine
7.2.1.1. six-membered heterocyclic aromatic rings containing two nitrogen at- oms
7.2.1.1.1. cytosine C
7.2.1.1.2. uracil U
7.2.1.1.3. thymine T
7.2.1.2. http://study.com/cimages/multimages/16/pyrimidine_group_600.png
7.2.1.3. pKa = how much it can hold on to its HYDROGEN
7.2.1.3.1. more basic = keto form
7.2.1.3.2. more acidic = enol/enamine form
7.2.1.3.3. important in determining whether these nitrogens serve as H-bond donors or acceptors.
7.2.2. purine
7.2.2.1. two rings of atoms: one resembling the pyrimi- dine ring and another resembling the imidazole ring
7.2.2.1.1. adenine A
7.2.2.1.2. guanine G
7.2.2.1.3. http://figures.boundless.com/11761/full/purines2.png
7.2.2.2. both have 1 NH at imidazole
7.2.3. http://www.atdbio.com/img/articles/purine-pyrimidine-large.png
7.2.4. Electron-Rich Nature
7.2.4.1. keto–enOL tautomeric shifts
7.2.4.2. keto tautomers of U, T, G vastly predominate at neutral pH
7.2.5. H-bond formation are the amino groups of cytosine, adenine, and guanine; the ring nitrogens at position 3 of pyrimidines and position 1 of purines; and the strongly electronegative oxygen atoms attached at position 4 of uracil and thymine, position 2 of cytosine, and position 6 of guanine
7.2.6. pKa = how much it can hold on to its HYDROGEN
7.2.6.1. more basic = keto form
7.2.6.2. more acidic = enol/enamine form
7.2.6.3. important in determining whether these nitrogens serve as H-bond donors or acceptors.
7.2.7. H-bond formation groups
7.2.7.1. NH2 groups of cytosine, adenine, and guanine
7.2.7.2. ring nitrogens at position 3 of pyrimidines and position 1 of purines
7.2.7.3. strongly electronegative oxygen atoms attached at position 4 of uracil and thymine, position 2 of cytosine, and position 6 of guanine
7.3. results when phosphoric acid is esterified to a sugar -OH group of a nucleo- side.
7.3.1. vast majority of monomeric nucleotides in the cell are ribonucleotides having
7.3.1.1. pKa value for the first dissociation of a proton from the phosphoric acid moiety is 1.0 or less, the nucleotides have acidic properties
7.4. Cyclic Nucleotides
7.4.1. Cyclic Phosphodiesters
7.4.1.1. phosphoric acid is esterified to two of the available ribose hydroxyl groups
7.5. Nucleoside Diphosphates (NDPs) and Triphosphates (NTPs) Are Nucleotides with Two or Three Phosphate Groups
7.5.1. formation of phosphoric anhydride linkages, as shown in Figure 10.13. Ad- dition of a second phosphate to AMP creates adenosine
7.5.1.1. Phosphate (Pi) + AMP (adenosine 5'-monophosphate)
7.5.1.1.1. Water + ADP (adenosine 5'-diphosphate) (reaction is a dehydration synthesis reaction.)
7.5.2. relatively strong polyprotic acids
7.5.2.1. dissociate three and four protons, respectively, from their phosphoric acid groups.
7.5.2.2. form stable complexes with divalent cations such as Mg2
7.5.2.2.1. NDPs and NTPs occur primarily as Mg2
7.5.3. indispensable agents in metabolism because the phosphoric anhydride bonds they possess
7.5.3.1. phosphoryl or pyrophosphoryl group transfer
7.5.3.1.1. release of a phosphoryl group from an NTP to give an NDP
7.5.3.1.2. the release of a pyrophosphoryl group to give an NMP unit
7.5.3.1.3. the acceptance of a phosphoryl group by an NMP or an NDP to give an NDP or an NTP
7.5.4. Division of labor
7.5.4.1. ATP
7.5.4.1.1. primary nucleotide in central pathways of energy metabolism
7.5.4.2. GTP
7.5.4.2.1. used to drive protein synthesis.
7.5.4.3. CTP
7.5.4.3.1. essential for phospholipid synthesis
7.5.4.4. UTP
7.5.4.4.1. forms activated intermediates with sugars that go on to serve as substrates in the biosynthesis of complex carbohydrates and polysaccharides
7.5.4.5. the four NTPs and their dNTP counterparts are the substrates for synthesis of the remaining great class of biomolecules—the nucleic acids.
7.6. Common ribonucleotides (mono)
7.6.1. Adenosine 5'-monophosphate (or AMP or adenylic acid)
7.6.2. Guanosine 5'-monophosphate (or GMP or guanylic acid)
7.6.3. CMP or cytidylic acid
7.6.4. UMP or uridylic acid
8. Ribonucleic acid (RNA)
8.1. RNA molecules are almost always single-stranded
8.2. Kinds
8.2.1. Messenger RNA Carries the Sequence Information for Synthesis of a Protein
8.2.1.1. mRNA molecules are transcribed copies of the protein-coding genetic units of DNA.
8.2.1.2. synthesized during transcription
8.2.1.2.1. Ribosomal RNA and tRNA molecules are also synthesized by transcription
8.2.1.2.2. an enzymatic process in which an RNA copy is made of the sequence of bases along one strand of DNA
8.2.2. Ribosomal RNA Provides the Structural and Functional Foundation for Ribosomes
8.2.2.1. Ribosomes, are about 65% RNA
8.2.2.1.1. Each subunit is a supramolecular as- sembly of proteins
8.2.2.1.2. Two subunits of different sizes that dissociate from each other if the Mg2+
8.2.2.2. fold into characteristic secondary structures as a consequence of intramolecular base-pairing
8.2.2.3. different species of rRNA
8.2.2.3.1. sedimentation coefficients
8.2.2.4. 80% of total CELLular RNA: represented by the various forms of rRNA.
8.2.2.5. rRNAs characteristically contain a number of specially modified nucleotides
8.2.2.5.1. pseudouridine residues, ribothymidylic acid, and methylated bases
8.2.3. Transfer RNAs Carry Amino Acids to Ribosomes for Use in Protein Synthesis
8.2.3.1. are small RNA molecules, containing 73 to 94 residues=
8.2.3.1.1. are methylated or otherwise unusually modified
8.2.3.1.2. All tRNA molecules possess a 3
8.2.3.2. Each AA has at least one unique tRNA species dedicated to chauffeuring its delivery
8.2.3.2.1. to ribosomes for insertion into growing polypeptide chains
8.2.3.3. Transfer RNA also has a complex second- ary structure due to many intrastrand hydrogen bonds.
8.2.4. Small Nuclear RNAs Mediate the Splicing of hnRNA into mRNA
8.2.4.1. Small Nuclear RNAs (snRNAs)
8.2.4.1.1. contain from 100 to about 200 nucleotides, some of which, like tRNA and rRNA, are methylated or otherwise modified
8.2.4.1.2. found in stable complexes with specific proteins forming small nuclear ribonucleoprotein particles (snRNPs)
8.2.5. Small RNAs Serve a Number of Roles, Including Gene Silencing
8.2.5.1. small RNAs, so-called because they are only 21 to 28 nucleotides long
8.2.5.1.1. noncoding RNAs
8.2.5.1.2. regulatory RNAs
8.2.5.1.3. direct readout.
8.2.5.2. Subclasses
8.2.5.2.1. small interfering RNAs (siRNAs)
8.2.5.2.2. micro RNAs (miRNAs)
8.2.5.2.3. small nucleolar RNAs (snoRNAs)
8.2.6. Long Noncoding RNAs (lincRNAs) Are Another Class of Regulatory RNAs
8.2.6.1. do not code for proteins
8.2.6.1.1. transcribed from DNA regions lying between genes
8.2.6.2. for regulation of chromatin structure and gene repression
9. DNA STRUCTURE
9.1. Det Primary Structure
9.1.1. type II restriction endonucleases
9.1.2. gel electrophoresis,
9.1.3. Chain termination/Dideoxy method by Sanger
9.1.3.1. Uses DNA Replication to Generate a Defined Set of Polynucleotide Fragments
9.1.3.1.1. DNA Replication
9.1.3.1.2. Chain Termination Protocol
9.1.4. ultra-high-throughput sequencing, or UHT, methods.
9.1.4.1. 454 Technology
9.1.4.1.1. relies on DNA polymerase-catalyzed copying of a primed single-stranded DNA
9.2. Secondary Structure
9.2.1. polynucleotide strands are inherently flexible
9.2.1.1. deoxyribose–phosphate segment of the backbone has six degrees of freedom
9.2.2. DNA Usually Occurs in the Form of Double-Stranded Molecules
9.2.2.1. ABZs of DNA secondary structure
9.2.2.2. General Features of DNA DOUBLE HELIX
9.2.2.2.1. a regular two-chain structure with hydrogen bonds formed between op- posing bases on the two chains
9.2.2.2.2. the polar sugar–phosphate backbones of the two chains are on the outside.
9.2.2.2.3. heterocyclic bases, as a consequence of their
9.2.2.2.4. Watson–Crick Base Pairs Have Virtually Identical Dimensions
9.2.2.2.5. Stability
9.2.2.2.6. OTHER CONFORMATIONS (that are not BDNA)
9.2.2.2.7. Dynamic feature
9.2.2.3. Secondary Structure of DNA Denaturation and Renaturation
9.2.2.3.1. denatured, and the strands separate as individual random coils
9.2.2.3.2. pH Extremes or Strong H-Bonding Solutes also Denature DNA Duplexe
9.2.2.3.3. Single-Stranded DNA CAN Renature to Form DNA Duplexes
9.2.3. DNA Adopt Structures of Higher Complexity
9.2.3.1. many DNA molecules are circular
9.2.3.1.1. bacterial chromosomes are covalently closed, circular DNA duplexes
9.2.3.2. Supercoils
9.2.3.2.1. two strands are wound about each other once every 10 bp or so
9.2.3.2.2. COMPACT the DNA
9.2.3.2.3. Basic parameter characterizing supercoiled DNA is the linking number (L)
9.2.3.3. Structure of Eukaryotic Chromosomes
9.2.3.3.1. 23 pairs of dsDNA molecules
9.2.3.3.2. SMC Proteins Establish Chromosome Organization and Mediate Chromosome Dynamics
9.3. Synthesizing Nucleic Acids Chemically
9.3.1. Phosphoramidite Chemistry Is Used to Form Oligonucleotides from Nucleotides
9.3.2. Genes Can Be Synthesized Chemically
10. Recombinant DNA: Cloning and Creation of Chimeric Genes
10.1. clone is a population of identical organisms derived from a single parental organism
10.2. Plasmids Are Very Useful in Cloning Genes
10.2.1. Plasmids are naturally occurring, circular, extrachromosomal DNA molecules
10.2.1.1. carry genes specify- ing novel metabolic activities
10.2.1.2. Restriction endonuclease digestion of plasmids can generate fragments with overlapping or “sticky” ends, artificial plasmids can be constructed
10.2.2. Plasmids as Cloning Vectors
10.2.2.1. cloning vectors (VEHICLES/CARRIERS) to carry genes
10.2.2.1.1. three common features of a cloning vector
10.3. Artificial Chromosomes Can Be Created from Recombinant DNA
10.3.1. 2 megabase pairs in length have been successfully propagated in yeast by creating yeast artificial chromosomes
10.3.1.1. including centro-telomeres
10.4. genomic library is prepared by isolating total DNA from the organism, digesting it into fragments of suit- able size, and cloning the fragments into an appropriate vector
10.4.1. Screening a genomic library by colony hybridization
10.4.1.1. Host bacteria transformed with a plasmid- based genomic library are plated on a petri plate and incubated overnight to allow bacterial colonies to form.
10.4.1.1.1. overlaying the plate with a flexible disc composed of absorbent material (such as nitrocellulose or nylon)
10.5. Virtually Any DNA Sequence Can Be Cloned
10.5.1. Nuclease cleavage at a restriction site opens, or linearizes, the circular plasmid so that a foreign DNA fragment can be in- serted
10.5.1.1. ends of this linearized plasmid are joined to the ends of the fragment so that the circle is closed again, creating a recombinant plasmid
10.5.1.1.1. Recombinant plasmids are hybrid DNA molecules consisting of plasmid DNA sequences plus inserted DNA elements (called inserts, chimeric constructs or chimeric plasmids)
10.6. cDNAs are DNA molecules copied from mRNA templates.
10.6.1. cDNA libraries are con- structed by synthesizing cDNA from purified cellular mRNA
10.7. Northern, Southern Blotting Hybridization
10.8. Reverse transcriptase
10.8.1. enzyme that synthesizes a DNA strand, copying RNA as the template
10.8.1.1. DNA polymerase is then used to copy the DNA strand and form a double- stranded (duplex DNA) molecule
10.8.1.1.1. Linkers are then added to the DNA duplexes ren- dered from the mRNA templates,
10.9. Expressed Sequence Tags
10.9.1. relatively short (
10.9.1.1. An EST represents part of a gene that is being expressed.
10.9.1.1.1. the probe
10.10. Expression vectors are engineered so that any cloned insert can be transcribed into RNA, and, in many instances, even translated into protein.
10.10.1. RNA Expression
10.10.2. ProteinExpression
10.10.2.1. cDNAs are DNA copies of mRNAs
10.10.2.1.1. cDNAs are uninterrupted copies of the exons of expressed genes
10.10.3. Screening cDNA Expression Libraries with Antibodies
10.10.4. Fusion Protein Expression
10.10.4.1. Translation of the recombinant sequence leads to synthesis of a hybrid protein or fusion protein.
10.10.4.1.1. N-terminal region of the fused protein represents amino acid sequences encoded in the vec- tor, whereas the remainder of the protein is encoded by the foreign insert
10.11. Polymerase Chain Reaction (PCR)
10.11.1. technique for dramatically amplifying the amount of a specific DNA segment
10.11.1.1. f denatured DNA containing the segment of interest serves as template for DNA polymerase
10.11.1.1.1. two specific oligonu- cleotides serve as primers for DNA synthesis
10.11.2. RT-PCR, a variation on the basic PCR method, is useful when the nucleic acid to be amplified is an RNA (
10.11.2.1. Reverse transcriptase (RT) is used to synthesize a cDNA strand complementary to the RNA, and this cDNA serves as the template for further cycles of PCR
10.12. In Vitro Mutagenesis
10.12.1. introduction of purposeful changes in the nucleotide sequence of a cloned gene represents an ideal way to make specific structural changes in a protein. The effects of these changes on the protein’s function can then be studied. Such changes constitute mutations introduced in vitro into the gene.
10.12.2. alter the nucleotide se- quence of a cloned gene systematically, as opposed to the chance occurrence of muta- tions in natural genes.
10.13. PCR-based mutagenesis
10.13.1. Mutant primers are added to a PCR reaction in which the gene (or segment of a gene) is under- going amplification
10.13.1.1. primers whose sequence has been specifi- cally altered to introduce a directed change at a particular place in the nucleotide se- quence of the gene being amplified
10.14. RNA interference
10.14.1. Inactivation of gene expression by RNAi is sometimes referred to as gene knockdown
10.14.1.1. introduction of double-stranded RNA (dsRNA) molecules into target cells by transfection, viral infec- tion, or artificial expression
10.14.1.1.1. Dicer is an RNase III family member that catalyzes endonucleolytic cleavage of both strands of dsRNA molecules to produce a double- stranded small interfering RNA
10.15. Retroviruses are RNA viruses that replicate their RNA genome by first making a DNA intermediate. Because retroviruses can transfer their genetic information di- rectly into the genome of host cells, retroviruses provide a route for permanent modi- fication of host cells ex vivo.
10.16. Human gene therapy seeks to repair the damage caused by a genetic deficiency through introduction of a functional version of the defective gene
11. DNA REPLICATION
11.1. Bidirectional
11.1.1. begins at one or more specific regions called the origin(s) of replication
11.1.1.1. bidirectional replication involves two replication forks that move in opposite directions.
11.2. Replication Requires Unwinding of the DNA Helix
11.2.1. For a double helix to unwind, it must either
11.2.1.1. rotate about its axis (while the ends of its strands are held fixed)
11.2.1.2. positive supercoils must be introduced
11.2.1.2.1. only choice when chromosome is circular
11.2.1.2.2. the unwinding reaction is driven by Helicases
11.3. Semidiscontinuous
11.3.1. both parental DNA strands are replicated at each advancing replication fork
11.3.1.1. DNA polymerase - carries out DNA replication
11.3.1.1.1. uses single-stranded DNA (ssDNA) as a template and makes a complementary strand by polymerizing deoxynucleotides in the order specified by their base pairing with bases in the template
11.3.1.1.2. synthesize DNA only in a 5
11.3.1.1.3. READSSS the antiparallel template strand in a 3
11.3.1.1.4. Replication at the 5’-3’ parent strand?
11.3.1.1.5. Functions:
11.3.1.1.6. can catalyze the synthesis of DNA in vitro if provided with all four deoxynucleoside-5
11.3.1.1.7. processivity: degree to which a particular DNA polymerase remains associated with the template through successive cycles of nucleotide addition
11.3.1.1.8. Types
11.4. DNA Replication Terminates at the Ter Region
11.4.1. Tus protein, to Ter
11.4.1.1. prevents the DNA duplex from unwinding by
11.4.1.1.1. blocking progression of the replication fork
11.4.1.1.2. inhibiting the ATP-dependent DnaB helicase activity
11.5. DNA Ligase Seals the Nicks Between Okazaki Fragments
11.6. The Cell Cycle Controls the Timing of DNA Replication
11.6.1. skip
11.7. How Are the Ends of Chromosomes Replicated?
11.7.1. ends of chromosomes have specialized struc- tures known as telomeres
11.7.1.1. short (5–8 bp), tandemly repeated, GC-rich nucleotide sequences that form pro- tective caps 1 to 12 kbp long at the ends of chromosomal DNA
11.7.1.1.1. maintenance and stability by protecting against DNA degradation or rearrange- ment.
11.7.1.2. Telomeres are added to the ends of chromosomal DNA by an RNA-dependent DNA polymerase known as telomerase
11.7.1.2.1. Telomerase is a specialized reverse transcriptase containing a catalytic subunit, referred to as TERT, for TElomerase Reverse Transcriptase
11.7.1.2.2. also contains an RNA component that serves as the template for telomere synthesis, referred to vari- ously as RT, for RNA Template, or TER, for TEmplate-containing telomerase RNA
11.8. RNA replication
11.8.1. RNA viral chromosome
11.8.1.1. DNA intermediate
11.8.1.1.1. RNA viral chromosome
11.8.2. RNA viruses that replicate their RNA genomes via a DNA intermediate are classified as retroviruses
11.9. DNA Repair
11.9.1. DNA is the only molecule that, if damaged, is repaired by the cell
11.9.2. accurate repair is possible because the infor- mation content of duplex DNA is inherently redundant
11.9.3. DNA damage may arise from en- dogenous processes or from exogenous agents
11.9.3.1. Endogenous
11.9.3.1.1. chemical reactions
11.9.3.1.2. loss of bases due to cleavage of N-glycosidic bonds
11.9.3.2. Exogenous
11.9.3.2.1. UV-induced free-radical generation
11.9.3.2.2. crosslinking of adjacent pyrimidines
11.9.3.2.3. breakage of the polynucleotide backbone by ionizing radiation
11.9.3.2.4. base modifications through chemical reactions.
11.9.4. Direct reversal repair systems
11.9.4.1. Chemical reactions that reverse the damage, returning the DNA to its proper state
11.9.4.1.1. i.e. methyltransferases
11.9.4.2. Single-strand damage repair
11.9.4.2.1. relies on the intact complementary strand to guide repair
11.9.4.3. double- stranded break (DSB) repair
11.9.4.3.1. particular threat to genome stability, lost sequence information cannot be recovered from the same DNA double helix
11.9.4.3.2. nonhomologous DNA end-joining, or NHEJ
11.9.4.4. translesion DNA synthesis
11.9.4.4.1. “error-prone” mode of replication allows the lesion to be bypassed
12. RNA Transcription Regulation
12.1. genes encoding the enzymes of a particular metabolic pathway are often grouped adjacent to one another
12.1.1. this pattern of organization allows expression in a coordinated fashion through transcription into a single polycistronic mRNA (single RNA transcript that encodes more than one polypeptide)
12.2. regulatory sequence-termed the operator
12.2.1. determines whether transcription takes place
12.2.2. next to a promoter
12.2.2.1. gene cluster + operator + promoter = operons
12.2.2.1.1. Transcription of Operons Is Controlled by Induction and Repression
12.2.2.1.2. two classes of genes:
12.2.3. Regulatory protein with the operator controls transcription of gene cluster
12.2.3.1. HOW? controlling access of RNA polymerase to the promoter
12.2.4. can be a palindromic DNA se- quence
12.3. Negative and Positive Control Systems Are Fundamentally Different
12.3.1. Genes under negative control are transcribed unless they are turned off by the presence of a repressor protein
12.3.2. genes under positive control are expressed only if an active regulator protein is present
12.4. Three critical base-paired hairpins can form in this RNA: the 1
12.4.1. Operon Attenuation I