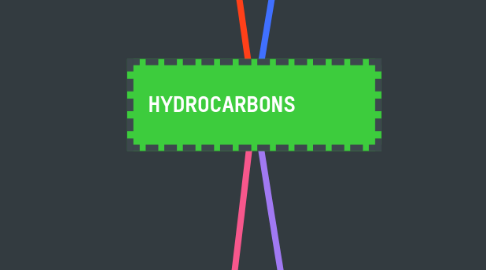
1. ALKANES
1.1. Preparation of Alkanes
1.1.1. By Clemmensen Reduction
1.1.1.1. R-CO-R/ R-CO-H + Zn-Hg/conc. HCl --> R-CH2-R/R-CH2-H + H2O
1.1.2. By Wolff Kishner reduction
1.1.2.1. R-CO-R/ R-CO-H + N2H4 + NaOH --> R/H-CH2-R
1.1.2.2. The Wolff-Kishner is done under strongly basic conditions using high heat in a polar protic solvent.The Clemmensen is performed in strongly acidic conditions. In both the reactions, reduction of ketone occurs.
1.1.3. By catalytic Hydrogenation
1.1.3.1. R-CH=CH2 + H2-Pd --> R-CH2-CH3
1.1.3.2. By Sabatier and Senderen Reaction y Here, catalysts used are Raney Ni [An alloy of Ni–Al] and Pd–Pt (Adam’s catalyst). y Temperature range suited for reaction is 300– 400°C. y In case of Raney Nickel reaction is possible at room temperature also.
1.1.4. Hydroboration reduction of alkene
1.1.4.1. R-CH=CH2 + BH3/THF + 3CH3COOH --> R-CH2-CH3
1.1.5. Wurtz reaction
1.1.5.1. 2(R-CH2-X) + 2Na/Dry ether --> R-CH2-CH2-R
1.1.5.2. In case of Wurtz reaction, alkyl halide is treated with sodium metal in presence of dry ether to give alkanes.Wurtz reaction is used to prepare alkanes containing even number of carbon atoms.
1.1.5.3. In case of tertiary–halide reaction is not possible as major product is according to dehydrohalogenation which means reaction holds only for a 1° or 2° halide.
1.1.5.4. In place of Na, activated Cu, Ag, Fe can also be used. y It is good for symmetrical alkanes (C2H6, C4H10) and CH4 can not be obtained.
1.1.6. Corey House synthesis
1.1.6.1. RX + Li + CuI + R'X --> R-R'
1.1.7. Frankland reaction
1.1.7.1. 2RX + Zn --> R-R + ZnX2
1.1.8. Kolbe electrolysis
1.1.8.1. RCOONa (aq.) + H2O --> R-R + CO2 + H2 + NaOH
1.1.8.2. Sodium or Potassium Salt of monocarboxylic acid is electrolyzed to form alkane. If n → no. of C-atoms in salt of carboxylic acid, Then, no. of C-atoms in alkane → 2(n-1)
1.1.8.3. pH increases during reaction due to formation of strong bases (NaOH / KOH). y If two different salts of acid are used, then a mixture of three alkanes will be formed. y If we take a mixture of sodium acetate and sodium propanoate then ethane, propane, butane will be formed. E
1.1.8.4. The process involves both, ionic as well as free radical mechanism.
1.1.9. Ullmann reaction
1.1.9.1. 2RI + Cu --> R-R
1.1.10. By Red P + HI
1.1.10.1. R-COOH/ RCHO/ RCOR + HI --> R-CH3/ RCH2-R
1.1.10.2. Red P in HI is a reagent that is used to generate Nascent Hydrogen which further helps in reduction.
1.1.11. By action of water on Al & Be carbide
1.1.11.1. Al4C3 + 12H2O --> 3CH4 + 4Al(OH)3
1.1.12. By decarboxylation of sodium salts of fatty acids When R–COOH heated with Sodalime (4 : 1 Mixture of CaO + NaOH) alkane is formed. Here alkane has one C– atom less than R–COOH as one CO2 molecule is eliminated.
1.1.13. By decomposition of Grignard Reagent y A compound possessing an active hydrogen atom generally shows this reaction with Grignard reagent.
1.1.13.1. R–MgX + H2O -> R–H+Mg.X.OH
1.1.13.2. When a Grignard reagent reacts with water, a proton replaces the halogen, and the product is an alkane.
1.2. Reactions of alkanes
1.2.1. Halogenation
1.2.1.1. i) R-CH3 + Cl2/Br2 + sunlight --> R-CH2-Cl/ R-CH2-Br + HCl/HBr
1.2.1.2. ii) R-CH3 + I2/sunlight/HIO3 --> R-CH2-I + HI
1.2.1.3. Note : Flourination is very fast and explosive
1.2.2. Finkelstein reaction
1.2.2.1. R-X + NaI/acetone --> R-I + NaCl
1.2.3. Sulphonation
1.2.3.1. R-H + Fuming H2SO4 + △ --> R-SO3H + H2O
1.2.4. Nitration
1.2.4.1. R-H + fuming HNO3 + △ --> R-NO2 + H2O
1.2.5. Chlorosulphonation with SO2 & Cl2
1.2.5.1. R-H + 2SO2 + Cl2 --> R-SO2Cl + 2HCl
1.2.6. Isomerization
1.2.6.1. i) n-Alkanes on heating in the presence of anhd. aluminium chloride and HCl gas isomerise to branched chain alkanes. Eg. n-butane + Hcl + AlCl3 --> Isobutane
1.2.6.2. ii) Note : Alkanes with 1-3 carbons, methane (CH 4), ethane (C 2 H 6), and propane (C 3 H 8,) do not exist in isomeric forms because there is only one way to arrange the atoms in each formula so that each carbon atom has four bonds.
1.2.6.3. The process of isomerization is good for increasing the octane number.
1.2.7. Combustion
1.2.7.1. R-H + O2 --> H2O + CO2
1.2.7.2. Note : Heat of combustion proportional to no. of carbon atoms but inversely proportional to branching.
1.2.8. Aromatization
1.2.8.1. R-C6H13 + Cr2O3/ V2O5/ Mo2O3 --> R-Ph
1.2.8.2. This process which involves cyclization, isomerization and dehydrogenation is called aromatization.
1.2.9. Pyrolysis
1.2.9.1. Breaking of higher alkanes into lower alkanes/ alkene
1.2.9.2. Eg. 2(C2H6) + △ (500℃) --> CH2=CH2 + 2CH4 + 2H2
1.2.10. Rxn. with steam
1.2.10.1. CH4 + H2O + Ni + △ --> CO + 3H2
1.2.11. Chemical Properties of Alkanes Alkanes are non-reactive towards bases, acids, oxidizing agents etc. due to non-polar nature of C–C and C–H bonds and the inability to donate or accept electrons. Isoination of alkanes is generally not carried out to prepare RI as the reaction is highly endothermic and reversible. But is carried out in the presence of an oxidizing agent, such as HIO3, HgO and HNO3, as it removes the HI formed in the reaction.
1.2.12. ALKYLATION
1.2.12.1. In this, H-atom of an alkyl group is substituted by an alkyl group.
1.2.12.2. Baeyer's Strain Theory : When ring compounds are formed, the bonds deviate from normal positions which produces a condition of strain in the molecule.
1.2.13. Cracking, Isomerisation and Aromatization are widely used to increase octane rating of gasoline. Sulphonation, Nitration, Pyrolysis all occur with free radical mechanism.
1.3. Physical properties of alkanes
1.3.1. i) Compounds formed by C-C & C-H sigle bonds Bond length of C–C is 1.54 Å and that of C–H bond is 1.11 Å.Alkanes gives a C–C bond energy of 80 kcal per mole and for C–H bond it is calculated as 97 kcal per mole.
1.3.2. ii) General formula --> C(n)H(2n+2)
1.3.3. iii) DU = 0 (except in cycloalkanes)
1.3.4. iv) All the carbon atoms present in an alkane are sp3 hybridised.
1.3.5. v) Alkanes are generally non-polar molecules. Hence, alkanes are hydrophobic in nature, that is, alkanes are insoluble in water.
1.3.6. vi) The boiling point & melting point of alkanes increases with increasing molecular weight.
1.3.7. The main sources of alkanes include petroleum, natural gas and coal.
1.3.8. Alkanes are also termed as paraffins due to their almost inert nature or reduced activity.
1.3.9. Alkanes show chain, position, and conformational isomerism.
1.3.10. Liquefied Petroleum Gas (LPG) is composed of propane, butane, and isobutane.
1.3.11. Physical state
1.3.11.1. C1– C4 ——colourless gases C5 – C17——colourless liquids C18 and above are colourless waxy solids.
1.3.11.2. Density ∝ Molecular weight [CH4 < C2 H6 <]
1.3.11.3. All alkanes are lighter than water and their limiting density is 0.8g /cm3
1.3.11.4. Cyclo alkanes float on water as they are lighter than water
1.3.11.5. Alkanes are insoluble in polar solvents but soluble in non-polar solvents like CCl4 , ether etc.
1.3.11.6. Solubility µ inversely proportional to Mol.wt CH4 > C2 H6 > C3 H8 > C4 H10 >
1.3.11.7. Boiling point ∝ Surface area α Molecular wt. The trend as shown by alkanes in relation to boiling points is as given: normal > iso > neo. when, for each successive member, molecular formula differs by a (–CH2 –) group and subsequently boiling point differs by 20°C – 30°C.
1.3.11.8. Melting point (M.P.) of even number alkane > Melting point of odd number alkane, i.e., C15 < C16 > C17
1.3.11.9. In case of alkanes with even number of C– atom more than the next alkane having odd number of C–atom as more symmetrical and have closer packing which generally means more stronger Van der Waal forces. It is called alternation or oscillation effect.
2. ALKYNES
2.1. Preparation of Alkynes
2.1.1. Using carbides: Carbides on hydrolysis give alkynes as follows CaO + 3C + 1800 degree celcius --> CaC2 + 2H2O --> Ca(OH)2 + ACETYLENE Mg3C2 + 4H2O --> CH3CCH=2Mg(OH)
2.1.2. Berthelots synthesis 2C +H2 +Electric arc 1200degree celcius --> acetylene
2.1.3. From CH4 6CH4 + O2 1500degree clcius --> 2CH2=CH2 + 2CO + 10H2
2.1.4. By heating CHX3 with silver powder CHX3 + 6Ag + CHX3 ∆--> ACETYLENE + 6AgX
2.1.5. Kolbe’s method: Sodium or potassium maleate or fumerate on their aqueous salt electrolysis gives acetylene at anode. Here, solution is basic and pH > 7. Sodium maleate/sodium fumerate +electric --> acetylene + 2CO2 (AT ANODE) + 2KOH + H2 (AT CATHODE).
2.1.6. By alkylation of acetylene or terminal alkynes. Acetylene reacts explosively with air, therefore it is replaced by oil gas.
2.1.7. By elimination reaction: Here, dihalides undergo α, β-elimination reaction with NaNH2 or alc. KOH to give alkynes. FOR EXAMPLE CH2Br-CH2Br +2KOH ∆--> acetylene +2KBr + 2H20 The most frequent application of these procedures arein the preparation of terminal alkynes.
2.2. Reactions of alkynes
2.2.1. HYDROGENATION OF ALKYNE R-C≡C-R + H2 +Pd/Pt/Ni --> R-CH=CH-R + H2 +Pd/Pt/Ni --> R-CH2-CH2-R
2.2.1.1. The reaction takes place on the surface of Pt or Pd. Since the addition is twice, it is hard to see the syn addition.
2.2.1.2. Nickel boride (P-2) gives syn-addition. y When Na/NH3 is used, hydrogenation is antiaddition.
2.2.2. PARTIAL HYDROGENATION OF ALKYNE BY POISON CATALYST Lindlar’s catalyst (PdCO3/BaCO3 ) poisoned with quinoline or lead acetate is generally used for selective hydrogenation in preparation of alkene only
2.2.3. Reaction with Halogen R-C≡C-R + X2 ONE MOLAR + CCl4 -->RXC=CXR + X2 ONE MOLAR + CCl4 -->RX2C-CX2R
2.2.3.1. In this, addition is anti and reactivity order for Halogens is Cl2 > Br2 > I2
2.2.3.2. ANTI-ADDITION TAKES PLACE
2.2.3.3. Westrone and westrosol both are good industrial solvents for oils, fat and waxes. WESTRONE CHCl2-CHCl2 WESTROSOL CCl2=CHCl
2.2.4. Addition of HX to alkynes: Addition takes place according to Markonikov’s rule and antiMarkovnikov’s rule.
2.2.4.1. Addition of HX to alkyne yields vinyl halides and alkylidene dihalides.
2.2.4.2. Reactivity order is HI > HBr > HCl
2.2.4.3. If we use CH3COBr/alumina, the rate of reaction increases.
2.2.4.4. Addition of HCl takes place at triple bonded carbon atom instead of double bonded carbon atom, because of formation of a more stable conjugated diene product which is resonance stabilised.
2.2.5. Reaction with HCN ACETYLENE + Ba(CN)2 +HCN POLYMERISATION -> Orlon or acrylonitrile (synthetic fber) or poly vinyl cyanide
2.2.6. Reaction with HOX: Addition occurs according to Markonikov’s rule and here X+ is attacking reagent.
2.2.7. Hydration of alkynes : Alkynes give Carbonyl Compounds on hydration by 1% HgSO4 and di/ H2SO4 as follows. R-C≡C-R + Hg2+ water --> R-CH2-CO-R
2.2.7.1. It is called kucherov reaction.
2.2.7.2. What is the role of HgSO4 in the hydration of alkynes?
2.2.8. Reaction with Alcohol CH≡CH + CH3COOH + HgSO4 --> VINYL ACETATE Polymerization--> PVA polyvinyl acetate (adhesive)
2.2.9. Reaction with AsCl3 CH≡CH + AsCl3 --> LEWISITE
2.2.9.1. Lewisite (β-chloro vinyl dichloro arsine) is a poisonous gas which on inhalation causes sudden death. The antidote for it is British anti-Lewisite (BAL).
2.2.10. Hydroboration: Used for preparation of ketones from alkynes except from ethyne which gives acetaldehyde.
2.2.10.1. The terminal alkynes can also undergo hydroboration. This proceeds in an antiMarkovnikov way as well
2.2.10.2. R-C≡C-CH3 i)B2H6/THF ii) NaOH/H2O2 --> R-CH2-CO-CH3
2.2.11. Cyclic polymerization: In this, alkynes polymerize to produce aromatic compounds. ACETYLENE + Fe red hot tube --> BENZENE
2.2.12. Formation of heterocyclic compounds i) When C2H2 is passed over iron pyrite at 300°C, it reacts with sulphur atom of iron pyrite to give thiophene. ii)Ethyne on reaction with ammonia gives pyrrole.
2.3. Properties of alkynes
2.3.1. Alkynes have a general formula of (CnH2n–2). For example, CH≡CH Ethyne CH3–C≡CH Propyne CH3 –C≡C–CH3 Butyne-2
2.3.2. Alkynes show chain, positional, functional isomerism (with alkadienes).
2.3.3. All the terminal alkynes have weakly acidic nature and acidic nature decreases in following order: R–C≡H > R–CH=CH2 > R–CH3 CH≡CH > CH3 –C≡CH > CH3 –CH2 –C≡CH > ….
2.3.4. C2 H2 is a linear compound, which is poisonous and has garlic like smell due to the presence of impurities of AsH3 , PH3 , H2 S.C2 H2 acts as a dibasic acid with very strong bases like NH2- , CH3-
2.3.5. Alkynes are generally less reactive to electrophilic addition because greater activation energy is needed as formation of a less stable cyclic reaction intermediate species takes place.
2.3.6. Test of Terminal Alkynes i)Terminal alkynes on reaction with with ammonical silver nitrate (AgNO3 ) give a white precipitate of R–C≡CAg. ii) Alkynes give a red precipitate of R–C≡C.Cu with ammonical CuCl.
2.3.7. Decreasing solubility in H2O: Alkynes > Alkenes > Alkanes Alkynes are slightly more soluble in H2O because they are somewhat more polar.
2.3.8. Acetylene is colourless gas having garlic like smell and boiling point of –84°C. It is poisonous and lighter than air
2.3.9. Alkynes having more than 3 carbon atoms are liquid while those with more than 14 carbon atoms are solids.They are lighter than water
2.3.10. Alkynes have higher B.P., M.P. than corresponding alkanes, alkenes because of more close packed arrangement (Linear Structure) and stronger interaction.
2.3.11. Acidic Nature of terminal alkynes: They are very less acidic in nature and it decreases in them with increase of no. of C–atoms.Metal acetylides can be used to distinguish terminal alkynes from non-terminal alkynes or alkenes.
3. ALKENES
3.1. Properties and Preparation of alkenes
3.1.1. Elimination reactions
3.1.1.1. Types of Elimination rxn. :- i) E1 rxn ii) E2 rxn / beta elimination rxn iii) E1-CB rxn
3.1.1.2. Eg. CH3-CH2X + alc. KOH + △ --> CH2==CH2
3.1.2. E1 mechanism
3.1.2.1. ii) Carbocation intermediate is formed (RDS step).
3.1.2.2. iii) It is a two step process.
3.1.2.3. iv) Rate of E1 rxn = K [RX]
3.1.2.4. v) No kinetic isotopic effect present.
3.1.2.5. vi) Pseudo Ist order rxn.
3.1.2.6. vii) It is a unimolecular elimination rxn.
3.1.2.7. i) Base attacks at alpha hydrogen atom.
3.1.3. E2 mechanism
3.1.3.1. i) Base attacks at beta H atom.
3.1.3.2. ii) No carbocation intermediate is formed.
3.1.3.3. iii) Kinetic isotopic effect is present.
3.1.3.4. iv) 5 membered transition state is evolved.
3.1.3.5. v) Rate of E2 rxn depends upon stability of alkene product. Rate = K [RX][base]
3.1.3.6. vi) Anti-peryplanar and bimolecular rxn.
3.1.4. E1-CB mechanism
3.1.4.1. i) It is a two step mechanism. ii) Carbanion intermediate is formed. iii) It is unimolecular conjugated base rxn. iv) 2nd order rxn. v) Kinetic isotopic effect is observed. vi) Presence of electron withdrawing group inc. rate of E1-CB rxn.
3.1.5. Dehydration of alcohols
3.1.5.1. R-CH(OH)-CH3 + conc. H2SO4 + △ --> R-CH=CH2
3.1.5.2. Rate of rxn depends upon stability of alkene formed. Saytzeff pdt is formed.
3.1.6. Dehydrohalogenation of alkyl halides
3.1.6.1. CH3-CH(Cl)-CH3 + Alc. KOH --> CH3-CH=CH2
3.1.7. Dehydrohalogenation of vicinal halides
3.1.7.1. CH2(Br)-CH2(Br) + Zn --> CH2=CH2 + ZnBr2
3.1.8. Electrolysis of aq. soln. of Na salts of COOH acids/ Kolbe electrolysis
3.1.8.1. NaOOC-CH2-CH2-COONa (electrolysis) --> CH2=CH2
3.1.9. Cracking of Alkanes
3.1.9.1. CH3-CH3 + △(600℃) --> CH2=CH2 + H2
3.1.10. Partial hydrogenation of alkynes
3.1.10.1. R-C2-R + H2-Pd/BaSO4 --> R-CH=CH-R
3.2. Physical properties of alkenes
3.2.1. i) Physical state : • These double-bonded compounds are colourless and odourless in nature. • However, ethene is an exception because it is a colourless gas with a faintly sweet odour. • The first three members of the alkene group are gaseous in nature, the next fourteen members are liquids and the remaining alkenes are solids.
3.2.2. ii) Solubility : • The alkenes are insoluble in water due to their nonpolar characteristics. • But are completely soluble in nonpolar solvents such as benzene, ligroin, etc.
3.2.3. iii) Boiling point and Melting point : • The boiling/ melting points of the compounds increase as the number of carbon atoms in the compound increases. • The boiling point of straight-chain alkenes is more than branched-chain alkenes just as in alkanes. • Cis-isomer molecules have a lower melting point than trans- isomers as the molecules are packed in a U-bending shape.
3.2.4. iv) Polarity : Alkenes are weakly polar just like alkanes but are slightly more reactive than alkanes due to the presence of double bonds.
3.2.5. x) Alkenes are often regarded as OLEFINS . The word olefin means making oil. Lower members of the group give oily products on halogenation.
3.2.6. v) Unsaturated carbon atoms are sp2 hybridized having a trigonal planar geometry.C=C bond has length as 1.34 Å and bond energy is 143.1 kcal per moleCarbon–Hydrogen bond length is 1.1 Å and has bond energy 98.7 kcal per mole. Alkenes exhibit chain, ring chain, positional, functional, optical, and geometrical isomerism.
3.2.7. vi) The simplest alkene CH2=CH2 goes by common name Ethylene.
3.2.8. vii) Symmetrical alkenes > unsymmetrical alkene trans alkenes > cis alkenes.
3.2.9. viii) Test of Unsaturation 1. Alkenes decolourize Br2 in CCl4 or when in water. 2. They also decolourize 1% of alkaline KMnO4 (Baeyer’s Reagent). 3. Ozonolysis gives number and location of double bonds in alkene.
3.2.10. ix) Ease of dehydrohalogenation 3° > 2° > 1°. Ease of dehydrohalogenation : Iodides > Bromides >Chlorides
3.3. Reactions of Alkenes
3.3.1. Addn. of hydrogen halides
3.3.1.1. R-CH=CH2 + HX --> R-CHX-CH3
3.3.1.2. Note : Carbocation intermediate is formed.
3.3.2. Anti Markonikov addn.
3.3.2.1. When HBr is added to unsymmetrical alkenes in the presence of peroxide, 1-bromopropane is formed contrary to 2-bromopropane (according to Markovnikov’s rule). This reaction is better known as anti-Markovnikov addition or Kharash effect after the name of M. S. Kharash who first observed it.
3.3.2.2. Eg. CH3-CH=CH2 + HBr + R2O2 --> CH3-CH2-CH2Br
3.3.3. Free radical addn
3.3.3.1. The best known and most important use of free radical addition to alkenes is probably polymerization.
3.3.3.2. An anti-Markovnikov halogenation is the free-radical addition of hydrogen bromide to an alkene. Eg. CH3-CH=CH2 + HBr + R2O2 --> CH3-CH2-CH2Br
3.3.4. Addn. of water
3.3.4.1. • R-CH=CH2 + H3O+/ H2O/ dil. H2SO4 --> R-CH(OH)-CH3 • R-CH=CH2 + D⁺/H2O --> CH3-CH(OH)-CH2D • R-CH=CH2 + H⁺/D2O --> CH3-CH(OD)-CH3
3.3.4.2. Carbocation intermediate is formed.
3.3.5. Addn. of cold conc. H2SO4
3.3.5.1. Cold concentrated H2SO4 adds to alkenes in accordace with markovnikov's rule to form alkyl halogen sulphate by electrophilic addition reaction.
3.3.5.2. Eg. CH2=CH2 + cold conc. H2SO4 --> CH3-CH2-OSO2OH
3.3.6. Addn. of halogens
3.3.6.1. R-CH=CH-R + Br2/CCl4 --> R-CHBr-CHBr-R Note : Addn of halogens in presence of CCl4 is anti-addn.
3.3.7. Addn. of halogen in aq. soln.
3.3.7.1. R-CH=CH2 + Br2/H2O --> R-CH(OH)-CH2Br
3.3.7.2. Note : -OH will be attached to the carbon atom with less no. of H atom.
3.3.8. Oxymercuration Demercuration
3.3.8.1. R-CH=CH2 + i) Hg(OAc)2, H2O ii) NaBH4/OH¯ --> R-CH(OH)-CH3
3.3.8.2. This is Markovnikov’s addn of H2O without rearrangement.
3.3.9. Hydroboration Oxidation
3.3.9.1. R-CH=CH2 + i) B2H6/THF ii) H2O2/OH¯ --> R-CH2-CH2(OH)
3.3.9.2. This is a anti-markovnikov's addn rxn.
3.3.10. Hydroxylation of alkenes
3.3.10.1. R-CH=CH-R + cold dil. KMnO4,NaOH / OsO4,tBuO2H,NaOH --> R-CH2(OH)-CH2(OH)-R Note : • This is a syn addn. rxn. • Cold dil. KMnO4,NaOH is called Baeyer's reagent.
3.3.10.2. R-CH=CH-R + CF3COOOH,H2O / M-CPBA,H2O --> R-CH2(OH)-CH2(OH)-R Note : This is a anti addn. rxn.
3.3.11. Hydrogenation
3.3.11.1. • R-CH=CH2 + H2-Pd --> R-CH2-CH3 • CH2-CH-CH-CH2 + H2-Pd --> CH3-CH-CH-CH3
3.3.12. Oxidation by hot conc. KMnO4
3.3.12.1. R-CH=CH-R + Hot conc. KMnO4 --> 2(R-CH=O)
3.3.12.2. Here, hydroxylation occurs by using OsO4, pyridine or NaHSO3/H2O, 1% alkaline KMnO4 (Baeyer’s reagent). y On hydroxylation, all of these reagents give syn addition.
3.3.12.3. Stereochemistry of hydroxylation cis alkene + cis mode reagent + 1% alk.KMNO4 or OsO4 /NaHSO3--> meso product trans alkene + cis mode --> reagent racemic mixture
3.3.13. Ozonolysis
3.3.13.1. CH2=CH2 + i)03 ii) Zn + H2O --> 2HCHO THIS IS CALLED REDUCYTIVE OZONOLYSIS.
3.3.13.2. CH2=CH2 + i)O3 ii) H2O2 --> 2HCOOH THIS IS CALLED OXIDATIVE OZONOLYSIS. If decomposition is carried out by H2O2 and Zn/CH3COOH is not used then dicarbonyl compounds formed undergo further oxidation to give acids.
3.3.13.3. Terminal alkynes give HCOOH as one of the products which is further oxidized to CO2.
3.3.13.4. Ozonide intermediate is formed.
3.3.14. Addition of oxygen
3.3.14.1. Oxydation or hydroxylation y Here, hydroxylation occurs by using OsO4, pyridine or NaHSO3/H2O, 1% alkaline KMnO4 (Baeyer’s reagent). y On hydroxylation, all of these reagents give syn addition.
3.3.15. Polymerisation
3.3.15.1. Polymerization is a process through which a large number of monomer molecules react together to form a polymer.
3.3.15.2. General catalyst used for polymerization of olefins is Zeigler-Natta catalyst[TiCl4 + (Et)3 Al].
3.3.15.3. nCH2=CH2 +△ HIGH TEMP --> [-CH2CH2-]n POLYTHENE FORMATION
3.3.15.4. Shifting of double bond (centre of the chain) or migration of methyl group takes place when heated at 770–970K or when heated with a catalyst Al2(SO4)3 at 470–570K
3.3.15.5. Cyclic polymerization: In this, alkynes polymerize to produce aromatic compounds 3 CH2=CH2 + Fe RED HOT TUBE --> BENZENE C6H6
4. ARENES
4.1. AROMATIC COMPOUNDS
4.1.1. Aromatic word has been derived from Greek word Aroma which generally means pleasant smell. Benzene and compounds which resemble benzene in chemical properties are known as Benzenoid compounds or Aromatic compounds. Aromatic hydrocarbons or Arenes have a general formula CnH2n6y (By represents No. of benzene Rings). and n > 6. main sources are coaltar coal, Petroleum etc.
4.1.2. Aromatic Compounds which don’t contain benzene ring are called non-benezeniods
4.1.3. BENZENE
4.1.3.1. Benzene was discovered by Faraday in 1825
4.1.3.2. The structure of benzene was given by Kekule in 1865
4.1.3.3. In benzene, all of the carboncarbon bond lengths are equal. Therefore, the Kekule structure is an incorrect representation of benzene
4.1.3.4. Preparation of benzene on large is done using light oil fraction and by petroleum.
4.1.3.5. All aromatic compounds are non-polar in nature.
4.1.4. TOLUENE
4.1.4.1. Toluene is a colourless liquid.
4.1.4.2. It is used as a solvent and in synthesis of other compounds.
4.1.4.3. Its boiling point is 111°C.
4.1.4.4. It is not miscible in water.
4.1.4.5. Reactions due to benzene ring: In this, methyl group activates benzene ring, Further substitution reaction takes place at ortho and para positions and rate of substitution reaction is more fast than in benzene.
4.1.5. Aromaticity and Hunckel (4n + 2)Rule Aromaticity is defned as “An aromatic compound having a reasonably planar cyclic structure, with (4n + 2)π electron clouds and having unusual stablity because of delocalisation of π electron.
4.1.5.1. According to Huckels Rule, Any compound can be aromatic when: (1) It has a cyclic planar structure (Necessary for complete delocalisation of πe–)
4.1.5.1.1. 2) It has a conjugate system
4.1.5.1.2. (3) It has high value of resonance energy
4.1.5.1.3. (4) It has number of π electrons according to (4n + 2) rule that is, 2, 6, 10, 14, etc. (where n = Number of cyclic planar ring). In case any compound has πe– according to 4n rule it is Anti aromatic. e.g., (1) When n = 0, No. of πe– = 2
4.1.5.1.4. (1) It has a cyclic planar structure (Necessary for complete delocalisation of πe–)
4.1.6. Aromatic compounds are less reactive than alkenes and alkynes due to the delocalisation of electrons.
4.2. PETROLEUM
4.2.1. It is a dark coloured, viscous, fluorescent liquid which is a mixture of aliphatic hydrocarbons cycloalkanes or aromatic hydrocarbons.
4.2.2. Various theories given on the origin of petroleum are:
4.2.2.1. 1. Mendeleev’s carbide theory
4.2.2.2. 2. Engler theory
4.2.2.3. 3. Modern theory
4.2.3. Refining of petroleum is the separation of useful fractions of petroleum and the removal of various undesirable impurities. It is mainly carried out by fractional distillation of crude oil
4.2.4. LPG AND NATURAL GAS AND CNG
4.2.4.1. LPG (Liquefied petroleum gas): It is a mixture of propane and butane, main component being butane. LPG has a smell like ethanthiol (C2H5SH), which is known as ethyl mercapton. The smell helps in detection of leakage of LPG.
4.2.4.2. Natural gas: It has 80% methane, 10% C2H6 and 10% long chain hydrocarbons.
4.2.4.3. CNG(Compressed Natural Gas): It has 95% CH4, 5% C2H6, C3H8, N2, He and CO2. CNG is liquefied natural gas.
4.2.5. Knocking Knocking is metallic sound produced due to pre-ignition of fuel in cylinder of an internal combustion engine.
4.2.5.1. Following hydrocarbons are arranged in decreasing order of knocking produced in them: Straight chain alkanes > branched chain alkane > olefns > cycloalkenes > atomatic hydrocarbons Tetraethyl lead (TEL) is used as an anti-knocking agent. It has (C2H5)4Pb (63%), C2H4Br2 (26%), C2H4Cl2 (9%) anddyes (2%).
4.2.6. Octane number y Quality of automobile fuel is measured with respect to octane number. Hydrocarbon iso-octane (2,2,4-trimethyl pentane) which has good resistance to knocking even in the highest compression motors is arbitrarily given an octane number of 100; whereas n-heptane which knocks maximum is arbitrarily given the value of zero. Thus, higher octane number, better will be fuel.
4.2.6.1. Two standards have been chosen-n–Heptane has octane number 0 whereas 2, 2, 4–Trimethylpentane (isooctane) has been given an octane number 100.
4.2.6.2. The octane number of gasoline is defined as the percentage of iso-octane present in a mixture of iso-octane and n-heptane, when the mixture has the same knocking in the engine, as the gasoline under examination
4.2.6.3. Thus, a motor fuel is said to have an octane number of 80 when it is as good as a mixture of 20 % n-heptane and 80% of iso-octane.
4.2.6.4. Octane number can be increased by:
4.2.6.4.1. 1. increasing the percentage of branched or cycloalkanes
4.2.6.4.2. 2. by addition of BTX
4.2.6.4.3. 3. by addition of TEL
4.2.6.4.4. 4. by addition of power alcohol
4.2.7. Cetane number: It is the percentage of cetane (hexadecane) in a given diesel sample. For example, if a diesel sample contains 75% cetane and 25% α-methyl naphthalene, its cetane number is 75.
4.3. Properties of BENZENE
4.3.1. It is highly inflammable.
4.3.2. Freezing point of benzene is 5.5°C
4.3.3. Its boiling point is 80°C.
4.3.4. It is immiscible in water.
4.3.5. A good solvent for resin, rubber and fat
4.3.6. Benzene is lighter than water.
4.3.7. It is colourless volatile, liquid
4.3.8. Chemical Properties Benzene has three alternative double bonds, still, it doesn’t show usual addition reactions or test of unsaturation with bromine water or Baeyer’s reagent. It gives electrophilic substitution reaction due to phenomenon of resonance or complete delocalization of six π electrons.
4.4. CHEMICAL REACTIONS OF BENZENE
4.4.1. Addition reaction Hydrogenation C6H6 + 3H2 + Ni/200 DEGREE CELCIUS YC--> CLOHEXANE
4.4.1.1. DEGREE OF UNSATURATION DECREASED FROM 4 TO 1
4.4.1.2. In the presence of sunlight and and absence of halogen carriers, benzene show addition reactions.
4.4.1.3. Iodination of benzene is a very slow reversible process, Hence, it is carried out in presence of oxidants like HIO3 or HgO to keep a check HI formation.
4.4.2. Birch reduction (selective hydrogenation)
4.4.3. Ozonolysis: Benzene on ozonolysis produces 3 GLYOXALS
4.4.4. With chlorine: C6H6 on chlorination in presence of sunlight produces gammexane or 666 or g-Lindane, which is a famous insecticide
4.4.5. ELECTROPHILIC SUBSTITUTION REACTIONS ARE CHARACTERSTIC REACTIONS OF AROMATIC COMPUNDS
4.4.5.1. HALOGENATION
4.4.5.2. NITRATION
4.4.5.2.1. Nitration and sulphonation both occur by the formation of the a-complex.
4.4.5.3. SULPHONATION
4.4.5.4. Friedel–Crafts reaction: In this,alkylation or acylation reaction takes place in presence of catalysts like BF3, ZnCl2, anhyd. AlCl3, FeCl3, or SnCl4.
4.4.5.4.1. ALKYLATION
4.4.5.4.2. ACYLATION
4.4.5.4.3. In Friedel–Crafts reaction, electrophiles are R+, RC+O, C+H=NH, C+OCl, C+ONH2, C+O.
4.5. PREPARATION OF BENZENE
4.5.1. From light oil fraction : Light oil fraction of coal-tar consists benzene, xylene, toluene. This mixture on fractional distillation produces benzene as one of the product.
4.5.2. Using acetylene : On passing acetylene through red hot tube polymerizes into benzene.
4.5.3. Using phenol : Phenol on distillation with Zn dust gives benzene.
4.5.4. Using chlorobenzene : Chlorobenzene or halobenzene on reduction with alcoholic Na or Ni–Al alloy gives benzene
4.5.5. Using benzoic acid : Chlorobenzene or halobenzene on reduction with alcoholic Na or Ni– Al alloy gives benzene
4.5.6. Using benzene diazonium chloride : Benzene diazonium chloride on reaction with hypo phophorus acid produces benzene.
4.5.7. Using benzene sulphonic acid : Benzene sulphonic acid on treatment with steam produces benzene.