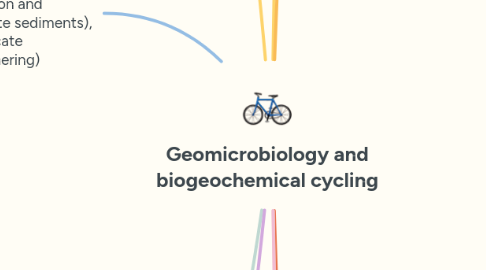
1. L4 (BGC) - **short - mid term** orgC cycle and the **long-term** organic C cycle
1.1. **short to mid term orgC cycle**
1.1.1. Biosphere to surface geosphere
1.1.2. transport **The marine biological pump** (orgC produced by photosynthesis in surface ocean and CO2 re-released in deep ocean via respiration)
1.1.2.1. CO2 conc depleted in surface ocean and increase with depth
1.1.2.1.1. but profiles differ between ocean basins as global conveyor belt parcel of deep water travels from Atlantic :arrow_right: Pacific accumulating released CO2 and nutrients along the way
1.1.2.2. oxygen minimum zones (OMZ) show the opposite trend from CO2 with O2 produced in the photic zone and consumed at depth (= biological pump) and regenerated at depth by cold O2 rich deepwaters = global conveyor belt
1.1.2.3. **The Redfield ratio**
1.1.2.3.1. marine phytoplankton incorporated nutrient elements into their tissues in specific ratios in the same ratio as seawater
1.1.2.3.2. nutrient profiles in seawater (concentrations are depleted in the surface and increase with depth, again due to the biological carbon pump which transports nutrients to depth, where they are released during respiration
1.1.2.3.3. Nutrients distributions in surface water. nutrients concentrations are lowest in open ocean waters and highest in upwelling regions
1.1.3. summary
1.2. **long term orgC cycle**
1.2.1. surface geosphere to deep geosphere
1.2.2. summary
1.2.3. **sedimentary orgC reservoir**
1.2.3.1. largest reservoir of orgC on earth
1.2.3.2. organic carbon leaks out of the short term carbon cycle (escapes respiration/decay) and is buried
1.2.3.3. occurs extensively with high sedimentation rates e.g., continental margins/low O2
1.2.3.4. e.g., orgc rich shales
1.2.3.5. Importance of OrgC burial to redox (allows O2 to build up in atmosphere and exerts control on global redox)
1.2.3.5.1. GOE - bury lots of organic carbon can release lots of oxygen - proved by C13 isotopes. carbon isotope record of marine carbonates increase correspond with GOE (gets heavier therefore burying more C12)
1.2.3.5.2. fraction of carbon buried as biomass vs organic
1.2.3.6. weathering of orgC in sedimentary rocks
1.2.3.6.1. after burial and lithification, sedimentary rocks enter the rock cycle- uplift and erosion
1.2.3.6.2. this is an oxidative process
1.2.3.6.3. the reverse of photosynthesis
2. L5 (BGC)- The **inorganic C** cycle- short term (CO2 to sea water), medium term (formation and deposition of carbonate sediments), long term (carbon-silicate cycle/burial and weathering)
2.1. inorganic/oxidised carbon reservoirs (CO2, carbonate)
2.2. **inorganic C cycle short term**
2.2.1. CO2 in seawater
2.2.1.1. CO2 dissolved in seawater and forms carbonic acid
2.2.1.2. this reaction can proceed backwards or forwards
2.2.1.3. equilibrium is reached quickly
2.2.1.3.1. the distribution of these forms of inorganic carbon in seawater is controlled by pH [H+] **bjerrum plot** - concentrations of the different carbonate species in solution as functions of pH
2.2.1.3.2. perturbation of this equilibrium ocean has a natural capacity to take up CO2 being released by the burning of fossil fuels **buffering capacity of the oceans**
2.2.1.4. carbonic acid dissociates rapidly at neutral pH, by losing protons (H+)
2.3. **inorganic C cycle medium term** (formation and deposition of carbonate sediments)
2.3.1. DIC reservoirs precipitation marine carbonates (depends on the saturation states of the fluids from which the carbonate is forming- depends on the calcium conc and carbonate conc)
2.3.1.1. due to increase fossil fuel burning there is more co2 dissolving into water which creates carbonic acid. carbonic acid likes to deprotonate into H+ and HCO3- ion. the small reservoir of CO3- in the ocean then absorbs the extra H+. this decreases the CO3- in the water and seawater becomes undersaturated in CO32- and then CaCO3 (corals) start to dissolve
2.3.2. **lysocline** and **calcium compensation depth (CCD)**
2.4. **inorganic C cycle long term** leaks (burial and weathering)
2.4.1. chemical weathering on land (carbonates and silicates)
2.4.1.1. CO2 dissolves in rainwater = carbonic acid which dissociates into H+ and HCO3-. rainwater doesn't have the buffering capacity (co3-) that the ocean has therefore rainwater is acidic
2.4.1.2. rocks undergo a chemical attack from this acid (carbonates CaCO3 and silicates CaSIO3)
2.4.1.3. **carbonate weathering**
2.4.1.3.1. products of weathering get washed into rivers and transported to the oceans
2.4.1.3.2. net effect of carbonate weathering and biomin
2.4.1.4. **silicate weathering**
2.4.1.4.1. **net effect** of silicate weathering and biomin
3. L6 (BGC) - Global **nutrient (N & P) cycles**
3.1. nutrients "tag along" on global carbon cycle
3.2. N and P used in DNA, RNA, proteins and ATP
3.2.1. P is the ultimate limiting nutrient as it has no atmospheric component unlike N
3.2.2. ultimate source and sink of N = biotic ultimate source and sink of P = abiotic
3.3. compare and contrast the different sources and sinks of these elements
3.3.1. **nitrogen**
3.3.1.1. reservoirs
3.3.1.2. nitrogen cycle (atmosphere to biosphere)
3.3.1.2.1. INPUT: biological **N2 fixation** source of fixed N to the biosphere (prokaryotes only) converts N2 (atmosphere) to Norg done by Diazotrophs e.g., marine cyanobacteria, soil and rhizosphere bacteria **nitrogen atmosphere :arrow_right: biosphere**
3.3.1.2.2. atmosphere to biosphere
3.3.1.2.3. once in ocean gets transported in the **marine biological pump** in the **redfield ratio** in poo and is respired deeper in the water column (**remineralization**- oxidation of organic matter) release of **DIN**
3.3.1.2.4. nitrogen can be buried in sediments and sedimentary rocks
3.3.2. Phosphorus
3.3.2.1. reservoirs
3.3.2.2. P cycle
3.3.2.2.1. Phosphorus weathering is the most important source of P to the oceans P comes from the geosphere rather than atmosphere. **Therefore different than C and N as has no atmospheric form**
3.3.2.2.2. p weathered from rocks goes to surface ocean. p from surface ocean to deep ocean **marine biological pump** (i.e. nutrient uptake in the surface ocean and nutrient release in the deep ocean)- biotic sink of phosphorous
3.3.2.2.3. unlike N p is very particle reactive (DIP= particle reactive). therefore another way p gets transported from surface ocean to deep ocean is by **Fe shuttle** where phosphate binds strongly to fe and mn oxides in oxygenated waters and sinks - abiotic sink of P
3.3.2.2.4. p becomes buried
4. summative diagram of the nitrogen cycle
5. L1 (GM) - **Extremophiles** through earths history
5.1. life on Earth: A timeline
5.2. 3 domains of life
5.3. Extremophiles definition
5.3.1. many forms of life thrive in environments outside our range of ability - anoxic, salty, acidic, very hot or cold, poisonous, high or low pressure, radioactive... **extremo**
5.3.2. most single celled microbes are **classified by what they love** - i.e., **phile**
5.4. Life on earth dominated by extremophiles
5.4.1. **Hadean Earth** 4 Ga GHB oceans hot and probably acidic **hyperthermophiles**
5.4.1.1. Hyperthermophile origin of life? red = **hyperthermophiles** (super heat lover)
5.4.1.1.1. temp up to 110DC
5.4.1.1.2. growth temp has a range too cold- membranes and proteins freeze too hot - proteins denature (become unfolded and coaggulated)
5.4.1.1.3. Psychrophile thermophile **hyperthermophile**
5.4.1.1.4. therefore origin of life... hydrothermal vents?
5.4.2. **Archean Earth** 4.0-2.5Ga atmosphere without oxygen no UV protection temp 30-80 **acidophiles**
5.4.2.1. GOE (2.3Ga)
5.4.2.1.1. oxygenic photosynthesis
5.4.2.1.2. weathering
5.4.2.1.3. **pH**
5.4.2.1.4. **acidophiles**
5.4.3. **Proterozoic** 2.5-0.5 sulphide-rich continental shelves anoxygenic phototrophs
5.4.3.1. meso-proterozoic 1.8-0.7Ga
5.4.3.1.1. anoxygenic photosynthesis
5.4.3.2. Neoproterozoic 0.7-0.5
5.4.3.2.1. Snowball earth 3 global glaciations
6. L2 (GM) - Redox and Microbial metabolisms
6.1. 5 requirements for life
6.1.1. **stable environment**- temp, salinity, pH (varies for organisms- i.e., extremophiles)
6.1.2. **liquid water**
6.1.3. **nutrients**
6.1.4. **carbon source**
6.1.4.1. METABOLISM
6.1.5. **energy source**
6.2. METABOLIC CLASSIFICATION
6.2.1. energy source
6.2.1.1. sunlight
6.2.1.1.1. phototrophy
6.2.1.2. chemicals
6.2.1.2.1. chemotrophy
6.2.1.2.2. use electrochemical energy, produced by coupling electron flow between chemicals in the environment (redox) **reduced e- donor to oxidized e- acceptor**
6.2.2. carbon source
6.2.2.1. CO2
6.2.2.1.1. autotrophy
6.2.2.1.2. primary producers
6.2.2.2. organic carbon
6.2.2.2.1. heterotrophy
6.2.2.2.2. secondary consumers
6.3. photoautotrophy
6.3.1. = photosynthesis
6.3.2. PP
6.3.3. types
6.3.3.1. oxygenic photosynthesis
6.3.3.1.1. cyanobacteria
6.3.3.2. anoxygenic photosynthesis
6.3.3.2.1. uses reduced compounds (including sulfur and iron) as electron donors
6.4. chemotrophy
6.4.1. energy from **redox reactions** e- donors and electron acceptors (e.g., respiration glucose e- donor and oxygen e- acceptor)
6.4.1.1. oxidation-reduction reactions
6.4.1.1.1. involve the **transfer of electrons** from one substance to another
6.4.1.1.2. **oxidation** = Loss of electrons and oxidized compound has had e- removed and therefore can accept electrons
6.4.1.1.3. **reduction** = gain of electrons a reduced compound has extra e- to donate
6.4.1.1.4. oxidation state toolkit
6.4.1.1.5. **half reactions** since electrons are being transferred, every redox reaction has two halves
6.4.2. many sources of e- donors and acceptors
6.4.3. = **ELECTRON TOWER** eukaryotes = red (aerobic respiration) vs microbes = black (anaerobic respiration)- using things to respire i.e., glucose that isn't oxygen
6.4.3.1. shows **electron potentials** for e- donors and e- acceptors (electron potential = ability to gain electron)
6.4.3.1.1. substances vary in their tendency to become oxidized or reduces, this is expressed as the electron potential
6.4.3.1.2. **negative pE** = *donate e- * the more negative the stronger the donor
6.4.3.1.3. **positive pE** = *accept e- * the more positive the stronger the acceptor
6.4.3.1.4. A-D>0 yay! (**gibbs free energy**) the largest difference in pE = highest energy
6.4.3.2. how much energy a reaction produces = **difference in electron potential**
6.4.3.3. if donor pE<acceptor pE = energy for life!
6.4.3.4. chemoheterotrophy: aerobic vs anaerobic
6.4.4. **Gibbs free energy**
6.4.4.1. = change in free energy during a reaction at standard conditions +G = creation of energy :) **endergonic** -G = needs energy :( **exergonic**
6.4.4.1.1. Gibbs free energy related to electron potential :arrow_up: difference in pE = :arrow_up:Gibbs free energy
6.4.5. **chemoheterotrophy = respiration** (oxidation of organic carbon)
6.4.5.1. aerobic respiration requires O2 and produces the most energy
6.4.5.2. anaerobic respiration required does not require O2 but produces much less energy (only bacteria and archaea)
6.4.6. biochemical zonation in marine sediments
6.4.6.1. use of e- acceptor in organic carbon respiration controls the chemistry of sediments and porewaters
6.4.6.2. for respiration of organic carbon, the efficiency of electron acceptors = oxygen>nitrate>>Fe-oxides>sulfate
6.4.7. **chemoautotrophy**
6.4.7.1. uses inorganic chemicals
6.4.7.1.1. e.g., Fe-oxidation
6.4.7.1.2. e.g., sulfide-oxidation
7. L3 - (BGC) carbon fixation and respiration in the **short-term** organic C cycle
7.1. key terminology in earth system science
7.1.1. **system** = a set of interconnecting parts (or **components**) that function together as a complex whole
7.1.2. **components**
7.1.2.1. **reservoir** e.g., atmospheric CO2, marine phosphate
7.1.2.2. a physical or chemical characteristic (or **state**) of the system (e.g., T or P)
7.1.2.3. larger systems can be broken down into **subsystems** at various scales
7.1.2.3.1. e.g., subsystems of the earth system
7.1.3. **reservoirs, fluxes, residence time**
7.1.4. **feedbacks in the earth system**
7.1.5. **equilibrium and perturbations**
7.2. CONCEPTS :brain:
7.2.1. CONCEPT 1: FEEDBACKS
7.2.1.1. components of a system can be linked (i.e., one directly responds to another) = **coupling**
7.2.1.1.1. **positive coupling**
7.2.1.1.2. **negative coupling**
7.2.1.2. couplings change or respond to disturbances, or **perturbations** to produce **feedbacks** = self-perpetuating mechanism of change and response to that change
7.2.1.2.1. a **negative feedback** diminishes the effects of disturbance = maintain stability and equilibrium
7.2.1.2.2. a **positive feedback** enhances the perturbation = disequilibrium (drives the system further from equilibrium)
7.2.2. CONCEPT 2: EQUILIBRIUM
7.2.2.1. balance exists in most systems due to the feedbacks between various components
7.2.2.2. when a system is in balance = equilibrium (steady state).
7.2.2.2.1. when a system is in a stable equilibrium state, modest perturbations do not push the system out of equilibrium due to negative feedbacks
7.2.2.3. equilibrium is maintained by feedbacks
7.2.3. CONCEPT 3: RESIDENCE TIME
7.2.3.1. the residence time of an element is the amount of time the element spends in a reservoir before it is removed
7.2.3.2. equation
7.3. short term organic C cycle
7.3.1. Reservoirs of carbon near Earth's surface (organic reduced and inorganic oxidised)
7.3.1.1. reservoirs are characterized in terms of the amount of material at any given time- mass or volume e.g., Gt C = 10(power 12)kg
7.3.1.2. inputs and outputs between reservoirs = fluxes e.g., (kg/yr) or (GtC/yr)
7.3.1.2.1. primary production (amount of orgC produced per unit time over a unit area of earth's surface) = **photoautotrophy** dominantly performed via **photosynthesis**
7.3.1.2.2. **chemoheterotrophy** performed by **aerobic respiration**
7.3.1.3. **residence time of CO2 in *atmosphere * **
7.3.1.3.1. system is in **equilibrium** input=output = steady state
7.3.1.3.2. seasonal imbalances photosynthesis> respiration (summer months) respiration>photosynthesis (winter months)
7.3.2. short term = atmosphere to biosphere