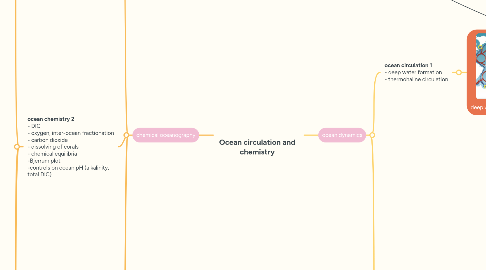
1. chemical oceanography
1.1. **ocean chemistry 1** - river water chem vs sea water chem - conservative, nutrient, scavenging - palaeoceanography
1.1.1. the composition of seawater is substantially different from river water reflecting additional inputs and outputs to seawater besides rock weathering
1.1.1.1. chemical compositions of natural waters rainwater is very pure and similar to seawater since it is derived from ocean aerosols. seawater has a very high TDS and is very different to river water
1.1.1.1.1. river water peaks = bicarbonate and calcium (from weathering of calcium carbonates)
1.1.1.2. sea water chemistry
1.1.2. residence times of dissolved constituents range <1000 - >100 million years
1.1.2.1. Conservative = millions of years nutrient = 100,000 years scavenged = 100s years
1.1.3. elements or ions exhibit conservative, nutrient or scavenging behaviour (or a combination of these)- classify the elements/ions in seawater based on their vertical profiles
1.1.3.1. **1. conservative**
1.1.3.1.1. the concentration of these elements/ions **normalized to salinity** is constant with depth and in the different oceans
1.1.3.1.2. most major seawater ions (Na+, Cl-) and non-major elements which usually have only weak biological reactions, are not particle reactive and are relatively soluble
1.1.3.2. **2. nutrient** INCLUDE ELEMENTS WHICH CAN SUBSTITUTE FOR MAJOR NUTRIENT ELEMENTS
1.1.3.2.1. the concentration of these elements/ions is controlled by biological cycling. profiles are depleted in surface waters and concentrations increase at depth due to decay and remineralization (**phytoplankton**)
1.1.3.2.2. as a deep water goes on its journey in the thermohaline circulation system it will **accumulate nutrients** therefore... NUTRIENTS CONCENTRATIONS INCREASE IN DEEP WATERS WITH DEEP WATER AGE= **INTEROCEAN FRACTIONATION**
1.1.3.2.3. Concentrations are lowest in open ocean waters (permanent stratification of water column) and highest in upwelling regions or in areas of coastal runoff
1.1.3.3. **3. scavenging**
1.1.3.3.1. these elements show some concentration decreases with depth
1.1.3.3.2. very particle reactive
1.1.3.4. **mixed behaviour**
1.1.3.4.1. e.g., Fe - has a nutrient type profile (consumed at the surface) but a residence time of 200-500 years (scavenged) and exhibits no inter-ocean fractionation (conservative) it is biologically essential and exhibits complex speciation in seawater speciation = distribution of an element among different chemical forms
1.1.4. the chemistry of marine biogenic carbonates may reflect past ocean chemistry
1.1.4.1. **palaeoceanography**
1.1.4.2. reconstructing past ocean nutrient conditions (N and P are not well preserved as it is incorporated into organic material but other nutrient type elements are such as caco3 as these are preserved in calcareous structures)
1.1.4.2.1. Ba and Cd can be substituted in CaCO3 crystal lattice and the Cd/Ca in carbonate reflects the Cd/Ca of seawater
1.1.4.2.2. also Ba/Ca increase seen in gulf of mexico which reflects barite used in offshore drilling
1.1.4.2.3. also use conservative type element (others = nutrient). Sr substitutes in place of Ca in aragonite lattice and is temp dependant therefore can construct past sea water temperatures
1.2. **ocean chemistry 2** - DIC - oxygen, inter-ocean fractionation - carbon dioxide - dissolving of corals - chemical equilibria -Bjerrum plot -controls on ocean pH (alkalinity, total DIC)
1.2.1. oxygen in seawater
1.2.1.1. DIC = high in surface waters, reaches a minima at 300-800m (OMZ) and increases at depth
1.2.1.1.1. high oxygen at surface = photosynthesis and contact with atmosphere means it can dissolve in
1.2.1.1.2. begin to increase respiration when leave photic zone until hit **compensation point** where photosynthesis and respiration balance
1.2.1.1.3. then respiration increases = **OMZ** associated with the decay of sinking particulate matter
1.2.1.1.4. oxygen increases again at depth due to the input of cold ( and therefore oxygen rich) deep waters which formed at the poles
1.2.1.2. more soluble at lower temperatures. this explains DIC in surface waters globally
1.2.1.3. on the deep water journey **oxygen decreases** as organic matter drops down and gets respired (which uses oxygen) = **inter ocean fractionation**
1.2.1.3.1. OMZ very pronounced at the tropics due to very well stratified water which inhibits the upwelling of water rich cool deep waters
1.2.1.3.2. i.e., atlantic> pacific DIC
1.2.1.4. **how does climate change impact dissolved oxygen**
1.2.1.4.1. expansion of oxygen minima zone (OMZ) with climate change in atlantic and pacific
1.2.2. **carbon dioxide in seawater** increasing atmospheric CO2 increases seawater DIC and HCO3- and H+ i.e., decreases pH . this decreased CO32- i.e., becomes undersaturated in water and causes corals to dissolve
1.2.2.1. pH is decreasing across the entire globe due to increased CO2 in the atmosphere
1.2.2.2. dissolved carbon dissolved CO2 partitions between carbon species
1.2.2.2.1. increase CO2 in the atmosphere = increase CO2 in water. double = double this will react with water to form more bicarbonate and protons and some protons are going to react with carbonate to form more bicarbonate **therefore increasing co2 decreases ocean pH (increases amount of H+ ions) AND decreases the amount of CO32-**
1.2.2.3. chemical equilibria
1.2.2.3.1. e.g., make CO2 = A and increase A. this means you are increasing the denominator which means k becomes smaller. but this cant happen because k is a constant. this means that some of a must react with b to produce C + D and k can stay the same
1.2.2.3.2. equilibrium constants for the dissolved inorganic carbon system
1.2.2.4. CO2, HCO3- and CO32- = DIC of seawater
1.2.2.4.1. Bjerrum plot at ocean pH HCO3->CO32->>CO2 **as pH increases we get a lot less carbonate**
1.2.2.5. ocean pH controlled by
1.2.2.5.1. total DIC **(increases with increased CO2 - i.e., forcing more co2 into water)**
1.2.2.5.2. alkalinity = total amount of base in seawater (i.e., negatively charges species which can react with protons to remove them from solution) - carbonate and bicarbonate
1.2.3. increasing atmospheric CO2 decreases CO32-
1.3. **ocean chemistry 3** - pH in the deeper ocean and between oceans - distribution of sediment types in modern ocean -CaCO3 dissolution
1.3.1. CaCO3 ocean sediments are derived from planktonic and benthic organisms which produce calcite or aragonite structures
1.3.2. what happens to pH in the deeper ocean and between oceans
1.3.2.1. **MARINE SNOW**
1.3.2.2. interested in particles which contain carbon (will effect pH) i.e., remineralisation/dissolution of organic and CaCO3 particles
1.3.2.2.1. dissolution of organics produces co2 and decreases pH
1.3.2.2.2. dissolution of CaCO3 produces carbonate ion (base) and increases pH
1.3.2.3. change through ocean basins in north atlantic have **low DIC and low total alkalinity** to pacific **increase DIC and alkalinity** due to dissolution of material in water column **overall going from high to low pH**- the dissolution of organic material>base material**
1.3.2.4. PH profile in the ocean
1.3.2.4.1. highest in the surface water and decreases with depth because getting decay of organic material. and pH is lower in the pacific than the atlantic because the water is older and has had more time to accumulate carbon
1.3.2.5. distribution of sediment types in modern ocean
1.3.2.5.1. NA has more CaCO3 than north pacific biogenic component from calcareous organisms e.g., foraminifera, pteropods, coccolithophores and siliceous organisms such as diatoms, radiolarians
1.3.2.5.2. particle sources
1.3.2.5.3. **30% rule** deep ocean sediment = >30% biogenic = calcareous or siliceous ooze deep ocean sediment = <30% biogenic = red clay
1.3.2.5.4. Carbonate becomes less common as travel from North Atlantic though indian ocean to south pacific to north pacific (**red clay does not become more abundant but carbonate becomes less abundant**)
1.3.3. CaCO3 dissolution is largely governed by seawater (CO32-)
1.3.3.1. equilibrium constants and dissolving minerals
1.3.3.1.1. Ksp = the product of the concentrations of the dissolution solutes at **equilibrium**
1.3.3.1.2. ion activity product (IAP) = the product of the activates of the dissolution solutes **in a sample**
1.3.3.2. solubility constants for aragonite and calcite
1.3.3.2.1. you can get a larger number for the moles of Ca2+ and CO32- in solution for aragonite and therefore it is more soluble (more ions in solution)
1.3.3.3. **summary diagram** for how does CaCO3 preservation change with depth and between oceans
1.3.3.3.1. Ca2+ = conservative type element CO32- (pH decreases down through the water column therefore CO32- will decrease)
1.3.3.3.2. adding CO2 therefore water gradually becoming more acidic
1.3.3.3.3. if CaCO3 is going to dissolve we want a low IAP (solution) **carbonate concentration in solution is less in the pacific** because pH = low therefore H+ ions react with CO32- this means the solution will become undersaturated with respect to the mineral and dissolve!
1.3.4. CO32- decreases from the N atlantic to the N pacific and as ocean depth increases so preservation of calcareous sediment becomes less likely
1.3.5. aragonite is more easily dissolved than calcite
1.4. **ocean chemistry 4** - areas we need to worry most about ocean acidification are the cold areas
1.4.1. ocean acidification is decreasing seawater CO32- This facilitates CaCO3 dissolution and hampers CaCO3 precipitation
1.4.1.1. how does increasing atmospheric CO2 affect the formation of marine CaCO3
1.4.1.2. decreases in sea surface pH are occurring globally, highest at the high latitudes (these are the parts of the ocean that are coldest and therefore where CO2 will most readily dissolve)
1.4.2. aragonite is more easily dissolved than calcite
1.4.3. many calcareous organisms increase the pH of the fluid used for calcification so may partially offset the impact of ocean acidification. the long term capacity of these mechanisms is unclear
1.4.3.1. how organisms produce CaCO3 structures (grown from a type of seawater)- if seawater is our starting solution for building the mineral (aragonite/calcite) how then will ocean acidification affect the solution which is used to build the mineral
1.4.3.1.1. look at the pH of the fluid at the calcification site - much higher than in seawater (therefore the animal is able to increase the pH) this increases the amount of carbonate in solution and therefore helps the organisms to build the mineral
1.4.3.1.2. even though calcification remained the same for one of these coral species as CO2 changes. the pH of the calcification sites varies dramatically
1.4.3.1.3. under ocean acidification we decrease the amount of carbonate in sea water meaning omega < 1 and undersaturated so the coral will dissolve AND the coral has more aspartic acid in the skeleton which prohibits the precipitation of the mineral
1.4.4. how does temperature, salinity and depth affect CO2 solubility
1.4.4.1. CO2 is more soluble at low temperature
1.4.4.2. CO2 is more soluble when the pressure is high
1.4.4.3. low salinity CO2 is more soluble
1.4.4.4. at high temperatures (CO2 less soluble) and low pressure (CO2 less soluble) favours carbonate over HCO3- therefore less ocean acidification
1.4.4.4.1. therefore the areas where we need to worry about ocean acidification are the areas that are cold (OMEGA LESS THAN ONE = UNDERSATURATED AND WILL DISSOLVE)
2. ocean dynamics
2.1. **ocean circulation 1** - deep water formation - thermohaline circulation
2.1.1. deep water formation (yellow sites)
2.1.1.1. must be dense (temperature and salinity)= **thermohaline circulation** (meridional)
2.1.1.1.1. deep water formed in antarcitc = **antarctic bottom water** (AABW)
2.1.1.1.2. **north atlantic deep water** (NADW)
2.1.1.1.3. temp and salinity = **conservative properties** of seawater therefore can be used as fingerprints
2.1.1.1.4. **antarctic intermediate water** (AAIW)
2.1.1.1.5. **Mediterranean sea** seen on deep water atlantic profile (very salty but warm water)
2.1.1.2. water formed in AABW travels into the Indian ocean and Pacific oceans
2.1.1.2.1. eventually mixing to become less dense i.e., in pacific (waters shear (mix)) and is replaced with fresh deep water which is coming in behind it
2.1.1.2.2. the oldest waters which are coming up in the Pacific formed as NADW 1000 years ago
2.1.1.3. NO DEEP WATER FORMATION IN THE PACIFIC but **pacific intermediate water** can just about make out some fingerprints
2.2. **ocean circulation 2** - surface currents; Coriolis force, Ekman spiral, gyers, pressure gradient and corilois force, geostrophic balance/current, westward intensification, convergence/divergence, upwelling/downwelling
2.2.1. surface currents (wind driven)
2.2.1.1. wind driven by trade winds close to the equator and midlatitude westerlies further away going the opposite direction
2.2.1.2. **Coriolis force**
2.2.1.2.1. moving north south moving to a latitude which has a slower eastward velocity therefore pulled to the right and visa versa for object moving south
2.2.1.2.2. moving east/west wind moving at same latitude (therefore has the same rotational velocity) but gravity pulls it down to the **centre** of the planet not the same latitude
2.2.1.2.3. in the northern hemisphere the coriolis causes bodies which are not frictionally bound the earth to veer to the right. and in the southern hemisphere to the left
2.2.1.2.4. **Ekman spiral** as depth of water increases the ocean flow becomes increasingly perpendicular to the direction of the wind down to 100m. in shallow waters friction of the bottom of the ocean dominates and the water will tend to move in the direction of the wind.
2.2.1.2.5. **gyers** CW northern hemisphere ACW southern hemisphere
2.3. **ocean mixing and stratification** - stable/unstable - molecular diffusion/turbulent diffusion - salt fingering - drivers of vertical changes in sea water density
2.3.1. water column stable = resistant to mixing = stratified water column unstable = mix vertically = unstratified
2.3.2. **ocean mixing**
2.3.2.1. **molecular diffusion** = cm scale process
2.3.2.1.1. is temperature dependant
2.3.2.1.2. temperature and salinity in the ocean do not move at the same rate **temperature homogenised in the ocean quicker than salinity as can bump into other molecules and pass on energy as well as move around** = **double diffusion**
2.3.2.2. **turbulent diffusion** = chaotic flow with irregular fluctuations in speed and direction- mm to 100s km process
2.3.2.2.1. wind driven waves
2.3.2.2.2. vertical diffusion> horizontal diffusion
2.3.3. what drives vertical changes in sea water density = **temperature and salinity**
2.3.3.1. global **temperature** profile reflects balance of heat gain and loss (incoming solar radiation)
2.3.3.1.1. Seawater temperatures
2.3.3.2. global sea surface **salinity** most saline in the middle of ocean gyres
2.3.3.2.1. most saline in middle of ocean gyres fresh in north pacific (i.e., why there is no deep water formation)
2.3.3.3. away from the sea surface, temperatures and salinity are much more constant
2.3.3.3.1. lines of equal temperature = **isotherms**
2.3.3.3.2. lines of equal salinity = **isohalines**
2.3.3.4. temperature, salinity and density profile with depth
2.3.3.4.1. temperature profile with depth section where temperature changes very rapidly = **thermocline** at the top temperature shows very little variation with depth = **mixed surface layer**
2.3.3.4.2. salinity profiles with depth are much more variable (usually higher at surface due to enhanced evaporation etc) but profile is still similar to temperature depth profile (well mixed surface layer, a region of rapid salinity change **halocline**
2.3.3.4.3. seawater density profiles change depending on equator, subtropics, high latitude. section where density changes very rapidly = **pynocline**
2.3.4. what is the **impact of climate change on ocean stratification** and primary production? increased stratification due to warming of surface waters, more storms = more turbulent mixing
2.3.4.1. increased temperature = increase stratification and increased freshness from ice melt = increased in stratification