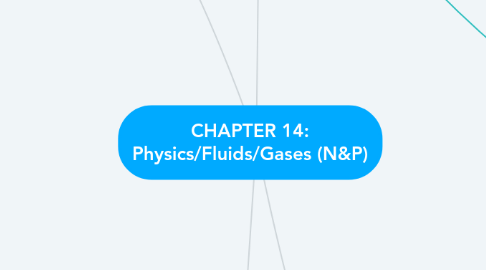
1. Measurement of Flow and Volume
1.1. Gas Volume
1.1.1. Respirometer
1.1.1.1. inspired Vt does not equal expired Vt
1.1.1.1.1. airway obstruction
1.1.1.1.2. apnea leak
1.1.1.1.3. disconnection
1.1.1.2. low Vt
1.1.1.2.1. mainstem intubation
1.1.1.3. high Vt or minute volume
1.1.1.3.1. vent failure
1.2. Equipment
1.2.1. Ventilators bellows scale
1.2.1.1. rough Vt
1.2.2. Wright spirometer
1.2.2.1. measure Vt
1.2.2.2. false high/low with high/low flow
1.2.2.3. small size, light weight but no alarms
1.2.3. Transduced Pneumotachograph
1.2.3.1. continuous recording of resp volume and air flow - measures rapid changes in respiration
1.3. Fick Principle - Caridac Output
1.3.1. if substance is added or removed from blood at known rate (O2/CO2), flow can be calculated if upstream and downstream concentrations are measured
1.3.1.1. Swan Ganz - indicator dilution (uses cold water to measure - temperature is indicator)
1.3.1.2. measures blood flow (CO) from difference in concentration of O2 or CO2 in arterial and venous blood
1.3.1.2.1. blood flow to organ = rate of arrival/departure of substance / difference of concentration of substance in arterial and venous blood
1.4. Gas Flows
1.4.1. Flow meter
1.4.1.1. Thorpe tube
1.4.1.1.1. flow increases when knob turned counterclockwise
1.4.1.1.2. low flow - opening is tubular, flow governed by viscosity
1.4.1.1.3. high flow - opening is orifice, flow governed by density
1.4.1.2. Leak in center flow meter - more O2 escapes than N2O = fatal
1.4.1.3. Flow meter indicator sequence can be cause of hypoxia
1.4.1.3.1. A & B potentially dangerous - N2O is downstream
1.4.1.3.2. O2 at nearest outlet - upstream leak results in N2O loss instead of O2, leaks downstream do not diminish delivered concentration, leaks distal result in volume loss w/o change in compositon
2. Fluids
2.1. solutions: A solution is a homogeneous mixture that consists of one or more solutes uniformly dispersed at the molecular or ionic level throughout a medium known as a solvent
2.1.1. homogenous mixture: not possible to discern phase boundaries between components of the mixture
2.1.1.1. NaCl
2.1.2. help chemical reactions
2.1.2.1. not necessarily liquid
2.1.3. solution forms
2.1.3.1. liquid in liquid
2.1.3.1.1. neostigmine in atropine
2.1.3.2. solid in liquid
2.1.3.2.1. sterile water in muscle relaxant
2.1.3.3. gas in gas
2.1.3.3.1. N2O in O2
2.1.3.4. gas in liquid
2.1.3.4.1. ABGs
2.1.3.5. amount of solute dissolvable in a given amount of solvent depends on temperature
2.1.4. solution states
2.1.4.1. unsaturated: permit more solute
2.1.4.2. saturated: no more solute without precipitation
2.1.4.3. supersaturated: heating saturated solution
2.1.5. Water: universal solvent
2.1.5.1. loses density as approaches 4C
2.1.5.1.1. 1gm/ml normally
2.1.5.1.2. Ice floats
2.1.5.1.3. expansion of water in cellular tissues = frost bite
2.1.5.2. molecule
2.1.5.2.1. V-shaped, 105 degree angle
2.1.5.2.2. O-H bonds are covalent
2.1.5.2.3. electrons are shared unequally with oxygen becoming slightly more negative and hydrogen becoming slightly more positive
2.1.5.3. Temperatures
2.1.5.3.1. FP
2.1.5.3.2. BP
2.2. movement through membranes
2.2.1. diffusion: movement of solute and solvent across a permeable membrane (or space in general)
2.2.1.1. high to low until equal
2.2.2. osmosis: movement of water across a semipermeable membrane to equilibrate a concentration gradient
2.2.2.1. osmotic pressure: stop osmosis
2.2.2.2. oncotic pressure: pressure by plasma proteins and e- on capillaries
2.2.2.2.1. 28 mmHg
2.2.3. tonicity of fluids: relative concentration of solutes in osmotic systems
2.2.3.1. isotonic: two fluids exert same osmotic pressure in opposite direction
2.2.3.1.1. 0.9% NaCl/ PRBCs
2.2.3.2. hypertonic: higher osmotic pressure than blood, higher solute (salt) load
2.2.3.2.1. crenation plasmolysis - draws water from the surrounding RBCs, causing them to shrink
2.2.3.2.2. 3% NaCl/RBCs
2.2.3.3. hypotonic: osmotic pressure less than that of blood
2.2.3.3.1. hemolysis: RBCs take on water and eventually rupture
2.3. Fluid Flow
2.3.1. F = Q/t : F (flow), Q (quantity - mass/volume), t (time)
2.3.1.1. Volume flow rate (Q): varies d/t cross sectional area, length, pressure diff, viscosity
2.3.2. Tube: pathway with length greater than diameter // Orifice: opening with diameter, no length
2.3.2.1. Types of Flow
2.3.2.1.1. Steady: uniform - molecules same direction, same velocity
2.3.2.1.2. Laminar- parallel
2.3.2.1.3. Turbulent - chaotic, irregular eddies
2.3.2.1.4. Transitional - laminar along walls, turbulent in center
2.3.3. Velocity: varies with cross sectional area of tube
2.3.3.1. Volume flow rate: varies with cross-sectional area of the tube, tube length, pressure differential, viscosity/density
2.3.3.2. F (volume flow or Q) determined by Poiseuille's Law
2.3.3.2.1. Poiseuille's Law: radius has effect on flow
2.3.4. Reynolds Number
2.3.4.1. ration of gas viscosity to density, predict flow velocity at which turbulence will occur
2.3.4.1.1. Re=vpd/n : v (linear velocity), p (density), d (diameter), n (viscosity)
2.3.4.2. directly proportional: velocity, density, diameter
2.3.4.2.1. *density determines flow, not viscosity
2.3.4.3. inversely proportional: viscosity
2.3.5. Bernoullis' Principle
2.3.5.1. fluid through tube containing constirction - pressure is least where flow is greatest
2.3.5.2. MDIs
2.3.5.3. Venturi Effect: steaming fluid regaining pressure higher than constriction requires tube distal to constriction to open gradually
2.3.5.3.1. nebulizer
2.3.6. Coanda Effect: fluid follows curve after constriction
2.3.7. Laplace's Law: all tension/pressure/radius in cylinder and spheres
2.3.7.1. cylinders - tension increased with inc radius (aneurysms)
2.3.7.2. surfactant
3. Physics
3.1. study of the behavior of atoms and the elements they make up
3.2. changes in matter d/t temperature, pressure, volume
3.3. Thermodynamics
3.3.1. 3 Laws: conservation, entropy, absolute 0
3.3.2. Energy: potential (PE= mgh), kinetic (KE=(1/2) mv2)
3.3.3. Entropy: move energy from high to low concentration
3.3.4. Temperature
3.3.4.1. measurement
3.3.4.1.1. Kelvin
3.3.4.1.2. Centigrade
3.3.4.1.3. Farenheit
3.3.4.2. Heat loss: High risk patients - higher BSA lower body fat, and elderly (unable to increase BMR for heat production)
3.3.4.2.1. Core temp redistribution: most in 1st hour*
3.3.4.2.2. Radiation (electormagnetic, greatest loss at head)
3.3.4.2.3. Convection (currents)
3.3.4.2.4. Conduction (contact)
3.3.4.2.5. Evaporation (humidity)
3.3.4.2.6. Volatile anesthetics cause vasodilation
3.3.4.3. Critical Temperature: point above which gas cannot be liquified
3.3.4.3.1. Critical Pressure: required to maintain liquid state (water 374C, 217mmHg)
3.3.5. Pressure
3.3.5.1. Kinetic Molecular Theory
3.3.5.1.1. molecules have no volume
3.3.5.1.2. no force unless collide
3.3.5.1.3. collisions on walls do not increase energy
3.3.5.1.4. molecules in constant random motion
3.3.5.1.5. temp depends on avg kinetic energy
3.3.5.1.6. speed (velocity), direction (vector), movement (energy) can vary
3.3.5.2. force exerted by collection of atoms or molecules, generated by 2 sources
3.3.5.2.1. gravity on atoms/molecules
3.3.5.2.2. energy sources within atoms/molecules
3.3.6. Volume
3.3.6.1. space occupied by aggregate or collection of molecules
3.3.6.1.1. solids: cm3, cc, or m3
3.3.6.1.2. liquids/gases: ml, L
3.3.6.1.3. IV flow, gas flow, tidal volumes
3.3.7. Vaporization: liquids with high vapor pressures at room temp - volatile liquids
3.3.7.1. isoflurane (238mmHg), Sevo (160mmHg), Des (660mmHg)
3.3.8. Thermal Expansion: increase in particle motion causes matter to expand, decrease = contract
3.3.8.1. Phases
3.3.8.1.1. solid
3.3.8.1.2. liquid
3.3.8.1.3. gas
3.3.9. DESFLURANE
3.3.9.1. saturated vapor pressure room temp (20C) =669mmHg (boiling at 23C)
3.3.9.2. TEC 6 Vaporizer
3.3.9.2.1. heated 39 degrees
3.3.9.2.2. 1550 mmHg
4. Gases: expand, compressible, high velocities (molecular activity), weak intermolecular forces
4.1. Empirical Gas Laws
4.1.1. Gas Properties: *exist in vacuum, no intermolecular forces*
4.1.1.1. Boyle: Pressure/Volume - inversly proportional (constant temp)
4.1.1.1.1. P1V1=P2V2
4.1.1.1.2. Ex: Measurement of Functional Residual Capacity (FRC-volume of gas in lung after normal expiration)
4.1.1.1.3. Ex. Ambu bag
4.1.1.1.4. Ex: normal tidal breathing
4.1.1.2. Gay-Lussac: Pressure/Temperature - directly proportional (volume constant)
4.1.1.2.1. P1T2=P2T1
4.1.1.2.2. N2O cylinder emptying - pressure decrease with liquid remaining (temp explained by Joule-Thompson, pressure by Gay-Lussac)
4.1.1.3. Charles: Temperature/Volume - directly proportional (constant pressure)
4.1.1.3.1. V1T2=V2T1
4.1.1.3.2. LMA cuff expands when autoclaved
4.1.2. Universal Gas Law (ideal) - volume for 1 mole will expand at given temp
4.1.2.1. PV=nRT
4.1.2.1.1. P=pressure, V=volume, n=moles, R=.0821, T=absolute Temp K
4.1.2.2. modified for real gases: P1V1T2=P2V2T1
4.1.2.2.1. volumes and pressures - same measurements
4.1.2.2.2. temperature converted to Kelvin
4.1.2.2.3. set up correct
4.1.3. Avogadro's Number: 1 mole = 6.0223x10 raised 23 at standard temp and pressure
4.1.3.1. volume = 22.4L
4.1.3.2. 1mole = 1g x molecular weight
4.1.4. Dalton's Law of Partial Pressures: multiple gases = % of total (P1 + P2 + P3...)
4.1.4.1. Partial pressure = concentration x barometric pressure
4.1.4.2. cylinder of compressed air (2000psi)
4.1.5. Adiabatic Changes: rapid expansion or compression of gas without equilibration - no increase or decrease in energy
4.1.5.1. Adiabatic heating: pressure on gas increases temperature
4.1.5.1.1. E cylinder O2 opened, O2 moves into a delivery tube between the cylinder valve and the diaphragm of a pressure gauge - increase in temp if heat not dissapated
4.1.5.2. Joule-Thompson Effect: gas under high pressure released into vacuum - rapid expansion (increase volume) lowers temp
4.1.5.2.1. soda can opening
4.1.5.2.2. E cylinder of pressurized O2 at 2000psi on back of anesthesia machine
4.2. Diffusion: gas ability to move from area of high concentration to low, eventual equilibration
4.2.1. Graham's Law: rate of effusion inversely proportional to square root of molecular weight
4.2.1.1. r=1/sq (mw): r (rate of diffusion), mw (molecular weight)
4.2.1.1.1. smaller mass = faster diffusion (greater velocity)
4.2.1.2. lighter gases diffuse faster than heavy gas
4.2.2. aplication
4.2.2.1. nitrous oxide
4.2.2.1.1. contraindicated with pneumo
4.2.2.1.2. ET cuffs cause tracheal mucosal damage
4.2.2.1.3. distention of bowel
4.2.3. Fick's Law: diffusion of gas across semipermeable membrane
4.2.3.1. Directly proportional to: partial pressure gradient, solubility of gas membrane, membrane surface area
4.2.3.2. Inversely proportional to: square root of molecular weight of gas, membrane thickness
4.2.3.3. Diffusion rate = (P1-P2)(Area)(Solubility)/ (membrane thickness) square (MW)
4.2.3.3.1. concentration effect: N2O diffuses out faster than N, gases left behind concentrated into smaller space
4.2.3.3.2. second gas effect: effective concentration of another agent increases - closer to inspired concentration
4.2.3.4. Pressure difference decreases as diffusion progresses (inhalation agent concentration as diffuses into blood stream)
4.2.3.4.1. gas exchange O2/CO2 across alveolar membrane
4.2.4. KNOW THIS!
4.2.4.1. CO2: larger than O2, diffuses 21x faster because lipid soluble
4.2.4.2. Equilibration of gas occurs when partial pressure is same everywhere
4.2.4.2.1. most likely will not reach in practical application due to variables
4.2.4.3. Diffuse of gas blood/alveoli requires difference in partial pressure
4.3. Solubility
4.3.1. Solids and Liquids
4.3.1.1. like dissolves like: salt and water
4.3.1.2. temperature: endothermic reactions (requires more energy than produced) increases solubility // exothermic, rare
4.3.2. Gases
4.3.2.1. Henry's Law: at constant temp gas dissolved in liquid directly proportional to partial pressure of gas at equilibrium above gas-liquid interface
4.3.2.1.1. solubility inversely related to temp - high temp = high kinetic energy, escaping molecules, low temp = retained molecules
4.3.2.1.2. p=kc: p (partial pressure), k (Henry's constant), c (concentration) -- increasing partial pressure of gas above liquid increases gas dissolved
4.3.2.1.3. calculate O2 and CO2 dissolved in blood