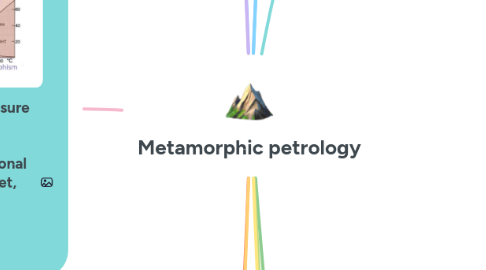
1. L7: high grade metamorphism and melting
1.1. metamorphism normally produces a fluid or a melt from 1. the breakdown of hydrous minerals or 2. warmer than normal conditions such as extension or magmatic zones
1.2. below the solidus fluid is produced (typically H2O and mixed H2O-CO2 rocks are also common) this includes internal and external buffering processes
1.3. **above the solidus** a melt is produced that is granitic in composition (this can also leave the source rock)
1.3.1. at T>=650 metapelitic and felsic water saturated rocks begin to melt metabasic rocks begin to melt at T>700
1.3.2. the **initial** melting reaction = **solidus**
1.3.3. On KFMASH
1.3.4. on facies diagram
1.4. occurs above the wet solidus for a granite and instead of a fluid (H2O) the rocks contain a granitic melt dominated by **anhydrous minerals** creating rocks called **migmatites** which are typically composed of distinct light and dark coloured minerals - where the melt faction is separated from the solid residual
1.4.1. high grade rocks have a weak foliation because they are poor in hydrous minerals (bi, mu, am) which typically define foliations this leaves them with a **granoblastic** texture. they are dominant in equant minerals such as pyroxene, plagioclase and garnet
1.4.2. **migmatite**
1.4.2.1. types of migmatite
1.4.2.1.1. **DIATEXITES** migmatites in which earlier metamorphic and protolithic features have been **destroyed or disrupted** look more like magmas than metamorphic rocks. may contain rafts of metatexites
1.4.2.1.2. **METATEXITES** migmatites in which earlier metamorphic and protolithic features have been largely preserved **(little disruption)** where evidence for bedding can be seen and form layered structures made up of alternating felsic and more ferromagnesian layers
1.4.2.2. parts of migmatite **paleosome- not melted** **neosome- layers affected by melting- leucosome (light coloured q and feld), melanosome/selvedge (dark coloured Fe-Mg minerals)**
1.4.2.2.1. e.g.,
1.4.2.3. textures
1.4.2.3.1. any melt in a high-grade rock has to crystallise as the rock cools- in the absence of deformation this may produce **igneous textures** if the melt has segregated into pools, may get typical metamorphic textures in areas of melt loss and igneous textures in melt pools
1.5. **typical high grade assemblages**
1.5.1. **Metapelites** muscovite replaced by K-feldspar bi + sill = gr +cordierite Bi = Orthopyroxene **THESE CHANGES ALL MAKE MELT**
1.5.1.1. THE REACTIONS THAT GOVERN THE DEVELOPMENT OF HIGH-GRADE MINERAL REACTIONS ARE THE REACTIONS THAT PRODUCE MELT AND PRODUCE THE SOLID MINERALS THAT TYPIFY THE GRANULITE FACIES **i.e., the reactions that produce melt also produce high grade assemblages**
1.5.1.1.1. the highest grade reactions don't produce that much new melt as don't involve hydrous minerals
1.5.1.1.2. the classic granulite facies assemblage g-cd-sill-opx-ksp
1.5.2. **Metabasites** any biotite breakdown sphere or epidote lost **amphi = clinopyroxene** am + bi = orthopyroxene gr appears **THESE CHANGES ALL MAKE MELT**
1.6. **melting and melting reactions**
1.6.1. melt = a new phase in the rock and forms via reactions
1.6.1.1. This melt is a complex hydrous silicate solution contains many other elements, notably the alkalis it is hydrophilic - needs water under metamorphic conditions exhibits exchange with minerals is a liquid product of reactions involving solids becomes less hydrous with increasing T
1.6.1.2. initially melt consumes H2O = **solidus reaction fluid present melting** where H2O fluid not present can form via the dehydration breakdown of hydrous minerals = **incongruent melting reaction**
1.6.2. **Fluid-present melting reactions** (wet solidus melting)
1.6.2.1. A + B + C... **+H2O** = liq (+D)
1.6.2.2. melt **consumes H2O**
1.6.3. ** incongruent melting** fluid-absent melting reaction/dehydration melting reaction
1.6.3.1. A + B ... +Dhyd = Liq + E
1.6.3.2. form **via the dehydration breakdown of hydrous minerals** (because most of the H2O in rocks is tied up in hydrous minerals, melting reactions that involve the breakdown of hydrous minerals are the most efficient at producing melt- 10-30% MELT
1.6.3.2.1. muscovite & biotite breakdown in metasediments amphibole breakdown in intermediate & mafic rocks
2. L6: fluids in metamorphism
2.1. metamorphism normally produces a fluid or melt from the breakdown of hydrous minerals (e.g., mica, chlorite, amphibole)
2.2. **below the solidus** the fluid produces is typically H2O (mixed H2O-CO2) rocks also common. there is internal and external buffering. fluid can leave the source rock.
2.2.1. minerals which carry OH- in their structure - when breakdown release OH as H2O. hydrous minerals include mica, chlorite, amphibole
2.2.1.1. when heat and deform a rock the total fluid content (minerals + porosity) decreases in both- but mostly in the minerals. and increases with grade
2.2.1.2. low grade assemblages are H2O rich and higher grade assemblages have less H20
2.2.1.3. rock has more water on prograde path than retrograde. it needs water added to form lower grade assemblages on the retrograde path
2.2.1.3.1. = **wet solidus** below the solidus hydrous minerals break down to release H2O. above the solidus they melt instead
2.2.1.3.2. leads to asymmetry of metamorphism- the retrograde history does not mean rocks go backwards through these reactions again so peak metamorphic minerals are preserved. due to dehydration reactions
2.2.1.3.3. **PRESERVATION VS RETROGRESSION**
2.2.2. fluid is a mobile phase - i.e., can enter or leave rock - an open system process - fluid is treated as just another phase in a rock but one that can move. can be a mixture and host ionic species and complexes
2.2.3. metamorphic fluids are low in salinity and dominated by H2O (pelites, metabasic rocks) and CO2(limestones,marls)
2.2.4. evidence of fluids = fluid inclusions, inferred from our understanding of processes, mineral equilibria can help predict fluid content and composition
2.2.5. **mixed fluids**
2.2.5.1. when fluid not just H2O the fluid-rock interaction is considerably more complex composition of the fluid becomes critical to mineral assemblage evolution most common mixed fluids are H2O-CO2 mixes
2.2.5.2. because we now have a **new compositional variable** 1. show effects of different fluid compositions in rocks 2. externally versus internally controlled equilibria 3. effect of different amounts of fluid interacting with rocks **versus if just H2O assemblage will be the same**
2.2.5.3. factors considered 1. composition of rock (hydrous minerals, carbonates) 2. composition of the fluid (Xco2 = CO2/(H2O + CO2)) 3. **amount of fluid influx** **lots = fluid composition controls equilibria (rock minerals)** **little = rock composition controls equilibria (fluid comp)**
2.2.5.3.1. **EXTERNAL BUFFERING** rock is subjected to an external infinite reservoir of fluid at a given Xco2 then the mineral assemblage and mineral compositions will change to those in equilibrium with that fluid
2.2.5.3.2. **INTERNAL BUFFERING** if the only fluid in a rock is that of the porosity, then changes in mineral assemblage, modes and compositions will control the composition of the fluid in the porosity
2.3. above the solids melt is produced which is granitic in composition. melt can leave the source rock.
3. L5: high pressure and low pressure environments note on diagram normal collisional metamorphism= barrow (garnet, sillimanite, kyanite) HTLP = buccan (cordierite, andalusite, sillimanite) LTHP=subduction(coesite)
3.1. although typical regional or Barrovian rocks dominate **can have more extreme metamorphism in different settings**
3.1.1. colder than normal **subduction zones** and early mountain building
3.1.1.1. **HPLT metamorphism**
3.1.1.1.1. interpreted as rapidly buried and exhumed rocks and therefore burial and exhumation rates are too fast for thermal equilibrium
3.1.1.1.2. contains ultra high pressure mineral such as **coesite** (high pressure quartz polymorph) and **microdiamonds**
3.1.1.1.3. P-T path = hairpin (rapid burial and fast exhumation)
3.1.1.2. **blueschist (glaucophane)-eclogite (omphacite) transition**
3.1.1.2.1. metamorphism of **arc systems** hydrated oceanic crust which is hydrothermally metamorphosed (zeolite and prehnite-pumpellyite) becomes blueschist then eclogite with increased pressure
3.1.1.2.2. amphibole (wet) :arrow_right: pyroxene (dry)
3.1.2. warmer than normal **extension/magmatic zones**
3.1.2.1. **LPHT metamorphism**
3.1.2.1.1. usually hot for depth. often similar minerals to contact metamorphism (contact aureoles) but are regional and deformed
3.1.2.1.2. **causes** 1. lithospheric thinning/loss of mantle lithosphere 2. magmatic heat 3. high internal heat production
3.1.2.2. **andalusite to sillimanite transition** (as opposed to ky-sill)
3.1.2.3. **buchan zones**
3.1.2.3.1. often **K-feldspar, Cordierite, Fe-spinel** (meta pelite)
3.1.2.3.2. biotite, cordierite, andalusite, sillimanite
3.1.2.3.3. another buchan sequence but going to granulite facies and **metabasic** (K-feld and ortho and clino pyroxenes)
3.1.2.3.4. Buchan vs Barrow
3.1.2.4. e.g., at high T (i.e., Bucchan) **pelite** = cordierite-garnet-sillimanite-K-feldspar-plagioclase-quartz **mafic** orthopyroxene-clinopyroxene-amphibole-plagioclase-quartz
3.1.2.5. most exstreme examples = Mt Stafford central-Aus where some melting occurs in the andalusite field. forming high Fe-spinel and cordierite
3.1.2.5.1. P-T
4. **METABASIC PETROGENETIC GRID**
4.1. AND FACIES
5. L8: metamorphic textures
5.1. the relationship between mineral shape and orientation tell us about timing
5.2. we use deformation events as time markers (similar to using fossils in sedimentary rocks). the relationship may be ambiguous because minerals take time to grow and foliations develop over time
5.3. the overall texture of the rock = fabric - composed of foliations, lineations, crenulations.
5.4. minerals
5.4.1. at the temperature/metamorphic grade increases so does the distance and rate of diffusion, so that crystals that form at higher temperatures are larger
5.4.2. earlier (prograde) minerals may be preserved as inclusions in peak minerals. later (retrograde) minerals may partially or completely replace peak minerals but retain the overall shape of the peak mineral = **pseudomorph** minerals may be **zones** reflecting changing P-T conditions. use metamorphic textures to unravel the **order of appearance of minerals**
5.4.3. **porphyroblasts** that overgrow the fabric during the prograde history e.g., if we have an eclogite facies rock that has porphyroblasts of garnet with inclusions of glaucophane and lawsonite= P-T path passed through blueschist facies
5.4.4. textures
5.4.4.1. **retrograde textures** common to get incomplete retrogression of peak phase = islands of peak phase surrounded in fine grained retrograde minerals reduction in temperature affects only edges of peak phases
5.4.4.2. foliations (mica, amphibole, quartz)
5.4.4.3. types of textures from contact metamorphism
5.4.4.4. replacement textures
5.4.4.4.1. hornblende partially replaced by glaucophane. retrograde texture and pseudomorph
5.4.4.4.2. garnet overgrown biotite in prograde texture
5.4.4.4.3. a corona
5.4.4.4.4. earlier muscovite foliation is crenulated (small folds) by a second deformation
5.4.4.4.5. inclusion trail oriented at an angle to the matrix foliation- helps to understand the timing of poikiloblast growth
5.4.4.4.6. relationship between porphyroblasts and matrix foliation help us understand timing.
6. **L2 +3 explanation**
6.1. what is a phase diagram
6.1.1. pictorial representation of mineral relationships
6.1.2. relates the number of phases to the number of chemical components being considered
6.2. main types
6.2.1. petrogenetic grids
6.2.1.1. P-T(projections)= bundles of reaction lines
6.2.1.1.1. only show stable reactions
6.2.1.1.2. are specific to a given chemical system e.g., KFMASH
6.2.1.1.3. only involve specified minerals
6.2.1.1.4. provide the backbone for our understanding of reactions and mineral assemblage stability (used to creates compatibility diagrams)
6.2.1.1.5. show reactions for all possible bulk rocks in the specified system, does not specify is a reaction will be see by a given rock
6.2.1.2. This diagram shows the P-T projections show the invariant and univariant reactions regardless of composition. we therefore don't know if a reaction will be seen by a given rock (its composition may not allow it)
6.2.1.3. e.g., metapelite petrogenetic grid - KFMASH = metapelite
6.2.1.4. Mapping petrogenetic grids onto AFM compatibility diagrams to define a reaction
6.2.1.4.1. purple field
6.2.1.4.2. red field
6.2.2. compatibility diagrams
6.2.2.1. linear or triangular compositional diagrams
6.2.2.1.1. show stable assemblages
6.2.2.1.2. drawn for specific systems e.g., KFMASH (system= sum of components e.g., oxides)
6.2.2.1.3. Fixed P and T
6.2.2.1.4. shows what minerals are stable for different P-T conditions
6.2.2.1.5. the chemical system has to be simplified to three components via projecting from some minerals
6.2.2.2. what minerals are stable at different compositions at fixed T and P
6.2.2.3. AFM diagram
6.2.2.3.1. Stable mineral assemblage for metapelite at greenschist facies depending on composition
6.2.2.3.2. AFM projection- metapelites with various compositions
6.2.2.3.3. reactions on AFM projections
6.2.3. Pseudosections
6.2.3.1. diagrams showing mineral stability for specified rock compositions
6.2.3.1.1. shows the stability of mineral assemblages in a given rock composition
6.2.3.1.2. A slice through P-T-X
6.2.3.2. This diagram illustrates that the same pressure and temperature will generate different minerals in a rock depending on that rocks composition. a pseudosection will fix this composition
6.2.3.3. Pseudosections for variable compositions
6.2.3.3.1. e.g., can set P or T- can have one variable and set XFe
6.2.3.4. P-X and T-X pseudosections
6.3. the controlling rules
6.3.1. the phase rule
6.3.1.1. relates the number of phases to the number of chemical components being considered and the variance (DOF) of the system
6.3.1.1.1. V=C-P+2
6.3.1.2. example- AL2SiO5 system
6.3.1.3. KFMASH and high variance example
6.3.2. Schreinemakers rule
6.3.2.1. controls the topology (shape of diagrams)
6.3.2.2. No mineral assemblage can exist in a greater than 180 degree arc
6.3.2.3. a reaction that will stop at an invariant point
6.3.2.3.1. one end of a reaction that passes through an invariant point is stable the other is metastable
6.3.2.3.2. the metastable extension for a reaction that lacks a given phase lies between reactions that produce that phases
6.3.2.4. application to ice-water-steam P-T reaction
6.3.2.5. Application to ice-water-steam P-T grid
6.3.3. the multivariant boundary rule
6.3.3.1. controls topography
6.3.3.2. applies to compatibility diagrams and pseudosections
6.3.3.3. the variance across a boundary between two assemblages can only change by 1
6.3.3.3.1. KFMASH example
6.3.3.4. intersections of boundaries always involve 4 boundaries
6.3.3.4.1. KFMASH example
6.3.3.5. the variance change though a point is either 0 or 2
6.3.3.5.1. KFMASH example
6.3.3.6. applied to compatibility diagram
6.4. changes in assemblages, modes & mineral composition as P & T vary
6.4.1. mineral assemblage
6.4.1.1. new minerals become stable
6.4.1.1.1. basis of the facies system
6.4.1.2. old minerals disappear
6.4.2. mineral proportions/modes
6.4.2.1. within each assemblage mineral proportions change (increase mode/decrease mode)
6.4.3. mineral composition
6.4.3.1. minerals with solid solution change their composition with changing P-T
6.4.4. every rock has a unique assemblage-mode-composition relationship. the more similar the bulk composition the more similar the response to changes in P-T
6.4.4.1. different assemblages in different bulk rock composition
6.4.4.2. same assemblage (St g chl), same compositions, DIFFERENT MODES
6.4.4.3. varying composition and modes
6.4.5. every rock composition responds differently to changes in P-T
6.4.6. The AFM movie how stable mineral assemblage changes with increasing pressure
6.5. relating the phase diagrams
6.5.1. P-T grid = shows the univariant reaction but not whether it affects our rock
6.5.2. compatibility diagram = shows the mineral equilibria relationships at fixed P-T for a wide rand of compositions. need a number of diagrams to show change
6.5.3. P-T pseudosection = shows all the mineral equilibria relationships over a P-T range but only for one bulk rock composition
7. **L4 explanation**
7.1. regional metamorphism
7.1.1. most common type of metamorphism
7.1.2. characterised by both metamorphism and deformation
7.1.3. commonly associated with continental collision - associated with mountain building processes (orogenesis)
7.1.4. metamorphic evolution of rocks used to infer the tectonic setting
7.1.4.1. Facies diagram
7.1.4.1.1. the P-T conditions that the rocks encountered on their journey is called a P-T path
7.1.4.2. metamorphic evolution
7.1.4.2.1. prograde (buried and heated)
7.1.4.2.2. peak metamorphism (max temp)
7.1.4.2.3. retrograde (exhumed and cooled)
8. L1: Principles of metamorphism
8.1. change in P and T = new mineral growth and textures controlled by **thermodynamics**- mineral groups which are stable at different pressure and temperatures, these will try to form the lowest energy configuration
8.1.1. P= Force per unit area
8.1.2. involves transient geotherms (stable geotherm is typically 30DC/km)
8.1.3. **metamorphic assemblage** = minerals that appear to stably coexist in a rock
8.2. **metamorphic environments** include regional, contact, hydrothermal, impact and fault
8.3. metamorphism often associated with mountain building processes
8.4. we see metamorphic rocks because they are **exhumed** due to isostatic rebound (orogeny is a transient unstable state for the crust imposed by plate tectonics)- rebounding continuously to find a new **isostatic equilibrium** due to continuous **erosion**
8.5. increase in coarseness and decrease in water content with increasing metamorphic grade
8.6. **protolith**
8.6.1. sedimentary **Al, K**
8.6.1.1. metapelite (siltsone and mudstone)
8.6.1.2. metapsammite (arenite or sandstone)
8.6.1.3. **metapelitic minerals**
8.6.1.3.1. **metapelitic minerals**
8.6.1.4. **Regional Barrovian metamorphism of pelites**
8.6.1.5. **Buchan (high temperature low pressure) vs Barrovian (high pressure, high temperature) metamorphism**- upper and middle crust
8.6.1.5.1. Graph trajectories
8.6.1.5.2. mineral assemblage
8.6.2. igneous **Fe,Mg,Ca**
8.6.2.1. metabasic
8.6.2.2. metagranite
8.6.2.3. **Metabasic minerals**
8.6.3. mineral assemblage = protolith and metamorphic grade (i.e., at the same grade (PandT a metasedimentary rocks will have very different mineral assemblage to metabasic rock) due to their different original compositions
8.6.3.1. we can use a few common protoliths to chart the changes in in mineral assemblage for different P&T conditions
8.6.3.1.1. e.g., compositional plots
8.6.3.2. e.g., at high T (i.e., Buchhan zone) **pelite** = cordierite-garnet-sillimanite-K-feldspar-plagioclase-quartz **mafic** orthopyroxene-clinopyroxene-amphibole-plagioclase-quartz
8.7. **metamorphic facies controlled by P and T**
9. L2 + 3: graphical representation of metamorphism
9.1. **Types of phase diagram**
9.1.1. **Petrogenetic grids (P-T projections)** shows all the reactions that will occur in a model system e.g., KFMASH however some bulk compositions will not see those reactions- shows reactions **show invariant points and univariant reaction lines for all the bulk compositions in a model system** (P-T projection for KFMASH)
9.1.1.1. i.e., shows the invariant points and univariant reactions regardless of composition
9.1.2. **compatibility diagrams** show the mineral assemblages and ranges of mineral solid solutions at a *specified P and T* for all the bulk compositions in the model system (e.g., AFM diagram for KFMASH)
9.1.2.1. composition relationships at **fixed P-T** we see what sets of minerals are stable for **different compositions**
9.1.3. **pseudosections** shows the fields of stability of different equilibrium mineral assemblage for a single bulk rock composition- shows fields of different stable phase assemblages. P-T pseudosection in KFMASH for common pelite: Al2O3 = 41.89, MgO = 18.19, FeO = 27.29, and K2O = 12.63 (in mol%).
9.1.3.1. T-X pseudosection in KFMASH for a composition line along which FeO:MgO varies, with X = FeO/(FeO+MgO), and Al2O3 = 41.89, FeO + MgO = 45.48, and K2O = 12.63 (in mol%)
9.1.3.2. set composition
9.1.3.3. can also have P-X pseudo sections
9.2. **controlling rules**`
9.2.1. The phase rule
9.2.1.1. relates the number of phases to the number of chemical components being considered and the variance (DOF) of the system
9.2.1.2. P=C-V+2
9.2.1.2.1. Components = chemical building blocks normally the oxides e.g., Al2O3, FeO
9.2.1.2.2. system = chemical system being studied = sum of components e.g. KFMASH system
9.2.1.2.3. variance = DOF
9.2.1.3. max number of phases when v (DOF)=0 therefore max variance = P=C+2
9.2.2. schreinemakers rule
9.2.2.1. controls topology (shape) of diagrams
9.2.2.2. no mineral assemblage can exist in a greater than 180 degree arc and a reaction will stop at an invariant point
9.2.3. the multivariant boundary rule
9.2.3.1. controls topology
9.2.3.2. the variance across a boundary between two assemblages can only change by 1
9.2.3.3. the variance change through a point is either 0 or 2
9.3. **representing reactions and mineral assemblage stability**
9.3.1. petrogenetic grids - bundles of reactions specific to a given chemical system show reactions for **all** possible bulk rocks in the specified system
9.3.1.1. **metapelite grid** KFMASH system
9.3.2. compatibility diagrams- show possible stable assemblage
9.3.2.1. DRAWN FOR FIXED P-T
9.3.2.2. if we take a pelite and pulverise it, then analyse its composition then we can plot it onto an AFM diagram
9.3.2.3. we can see the relative proportions of biotite and chlorite
9.3.2.4. we can also represent reactions e.g., g + chl = bt +st
9.3.2.4.1. =
9.3.2.4.2. this includes moving across a reaction line in a P-T diagram
9.3.2.4.3. **crossing tie lines = reaction**
9.3.2.5. stable assemblage for these p-t ranges
9.3.3. pseudosections - diagrams drawn for specific rock compositions show all mineral equilibria relationships for **a given rock composition**
9.4. **changes in assemblages, modes & mineral composition as P&T vary**
9.4.1. mineral assemblages- new minerals become stable, old minerals disappear= bases of the facies system
9.4.2. within each assemblage mineral proportions change same assemblage different modes
9.4.3. minerals with solid solution change their composition
9.4.4. different assemblages in different bulk rocks
9.4.5. varying composition and modes
9.5. **relating phase diagrams**
9.5.1. T-X combines features from both compatibility diagrams and P-T pseudosections
10. **METAPELITE PETROGENETIC GRID** Petrogenetic grid for metapelites (click to zoom).[1][2] Each line represents a metamorphic reaction. Metamorphic facies included are: BS = Blueschist facies, EC = Eclogite facies, PP = Prehnite-Pumpellyite facies, GS = Greenschist facies, EA = Epidote-Amphibolite facies, AM = Amphibolite facies, GRA = Granulite facies, UHT = Ultra-High Temperature facies, HAE = Hornfels-Albite-Epidote facies, Hbl = Hornblende-Hornfels facies, HPX = Hornfels-Pyroxene Facies, San = Sanidinite facies
11. L4: Regional metamorphism and barrow zones
11.1. **regional metamorphism**
11.1.1. mostly due to crustal thickening due to continental collision i.e., continental collision
11.1.2. characterised by **metamorphism and deformation**
11.1.3. intermediate dP/dT conditions = gr-am-gr facies
11.1.4. clockwise P-T paths = burial-heating-exhumation
11.1.4.1. burial and heating= prograde metamorphism **max temp** = peak metamorphism (rocks are buried faster than they can thermally equilibrate) exhumed and cooled = retrograde metamorphism
11.1.4.2. P-T paths vary through an orogen
11.1.4.2.1. highest grade is in the middle of mountain belt which is eroded most therefore **walk downhill to higher grade rocks**
11.1.4.3. exhumation pathway depends on is erosional or extensional
11.1.4.4. ACW P-T paths tend to be dominated by a magmatic hear source early
11.1.5. **barrow zones**- common product of regional metamorphism, zones and isograds (field gradient)
11.1.5.1. **appearance** of key mineral mapped by **isograd**
11.1.5.2. chl-bi-gr-str-ky-sil (metapelites)
11.1.5.3. note not all ky rocks contain kyanite this is due to different compositions of rocks. therefore must use a sequence of similar rock compositions. for barrow zones this is low-med Al, high Fe
11.1.5.4. lateral variation in peak metamorphic minerals = field gradient
11.1.5.5. some barrow zones go to even higher grades than the scottish ones e.g., get cordierite
11.1.5.6. **how the barrow zones match up with facies diagrams** (N America leans more towards high temperature Bucchan especially with cordierite being present)
11.2. **metamorphism = asymmetric**
11.2.1. rocks release H20 as they metamorphose i.e., **dehydration reactions**
11.2.2. i.e., the rocks we see at the surface preserve the peak metamorphic minerals
11.3. **mineral assemblage evolution**
11.3.1. EACH ROCK WITH DIFFERENT STARTING ASSEMBLAGE ENDS UP WITH THE SAME ASSEMBLAGE BUT HAVE DIFFERENT EVOLUTIONS- WE WOULD RELY ON OBERVATIONS SUCH AS INCLUSIONS IN PRPHYRO-BASTS TO DISTINGUISH THE DIFFERENT EVOLUTIONS
11.3.1.1. COMPOSITIONS OF BULK ROCKS
11.3.1.2. MINERAL ASSEMBLAGE EVOLUTION