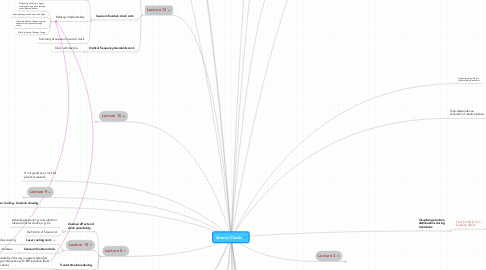
1. H not good as a clock for practical reasons.
2. Foot §9.1 - The scattering force.
2.1. Momentum transfer and scattering rate.
2.2. Balance of absorption and stimulated emission.
2.3. Maximum light force.
2.3.1. Maximum light acceleration.
2.4. Atom beam stopping distance.
3. Breit-Rabi diagram of H ground state hyperfine structure..
4. Laser cooling - Zeeman slowing
5. Transition least sensitive to magnetic fields.
6. Light forces.
7. Lecture 7
7.1. Zeeman effect.
7.1.1. Foot § 5.5 - Zeeman effect.
7.1.1.1. Zeeman effect in LS- coupling scheme.
7.1.2. ZE in weak magnetic field.
7.1.3. ZE in strong magnetic field.
7.1.4. Calculate Zeeman shifts of H ground state hyperfine levels using DPT.
8. Lecture 8
8.1. Zeeman effect and clock uncertainty.
8.1.1. Advantages to using 'one-electron' alkali atoms for clocks, e.g. Cs.
8.1.2. Definition of SI second.
8.2. Lecture 12
8.2.1. Laser cooling cont.
8.2.1.1. Sisyphus cooling
8.2.1.1.1. Recoil limit.
8.2.2. Caesium fountain clock.
8.2.2.1. Moving molasses.
8.3. Transit time broadening.
8.3.1. Probability of being in upper state after single interaction with EM radiation (Rabi equation).
8.3.1.1. Foot §7.1, §7.3 and additional notes.
8.3.1.2. pi-pulses and pi/2-pulses.
8.3.2. Longer cavity versus slower atoms.
8.3.2.1. Foot §6.4.2 - Atomic clocks.
8.4. Atomic beam technique
9. Lecture 9
9.1. Light forces cont.
9.1.1. Can a lamp be used as the photon source to stop an atom beam?
9.2. Light interacting with atoms.
9.3. Laser cooling.
9.3.1. Doppler shift.
9.3.1.1. Laser detuning.
10. Lecture 10
10.1. Laser cooling - optical molasses.
10.1.1. TIPT gets it wrong, but gives good insight.
10.1.1.1. Foot §7.4 - Ramsay fringes.
10.1.2. Foot §9.3 - The optical molasses technique.
10.1.2.1. Friction like velocity and lineshape dependent force.
10.1.2.2. Cooling rate.
10.2. Laser cooling - Doppler limit
10.2.1. Calculate Doppler limit temperature.
10.2.2. Calculate Doppler limit velocity.
10.2.3. Calculate recoil velocity.
10.2.4. Calculate capture velocity.
10.2.5. Foot §9.2 - Slowing an atomic beam.
10.2.6. Foot §9.7 - The Sisyphus cooling technique.
10.3. Optical frequency standards.
10.3.1. Clock frequency instability.
10.3.2. Requirements for optical frequency standard.
10.3.2.1. Foot §8.5.3 - Optical frequency combs.
11. Lecture 13
11.1. Lecture 16
11.1.1. 27Al+ ion quantum logic clock cont.
11.1.1.1. Quantum logic spectroscopy.
11.1.1.1.1. Pulse sequence for measuring transition frequency in 27Al+ ion.
11.1.2. 171Yb+ ion clock.
11.2. Caesium fountain clock cont.
11.2.1. Double pass through microwave cavity.
11.2.2. Ramsay interferometry.
11.2.2.1. Probability of being in upper state after single pass through cavity (Rabi equation).
11.2.2.2. Extra phase evolution from free flight.
11.2.2.3. Total probability of being in upper state after two passes through cavity.
11.2.2.4. Width of central Ramsay fringe.
11.2.3. Summary of caesium fountain clock.
11.3. Optical frequency standards cont.
11.3.1. Clock architecture.
12. Lecture 14
12.1. Ultra-stable laser.
12.1.1. Optical lattice clock.
12.1.1.1. Advantages over ion clocks.
12.1.2. Pound-Drever-Hall (PDH) locking to ultra-stable cavity.
12.2. Femtosecond frequency comb.
13. Lecture 15
13.1. Linear Paul trap for ions.
13.1.1. Earnshaws theorem - saddle point, need RF.
13.1.2. Stable ion trajectories in trap.
13.2. 27Al+ ion quantum logic clock.
13.2.1. Laser cooling to quantum harmonic oscillator.
13.2.2. Elimination of first order Doppler shift in Lamb-Dicke regime.
13.2.3. Why need for the 25Mg+ ion?
13.2.3.1. Sympathetic cooling and quantum logic spectroscopy detection of clock transition in 27Al+ ion.
14. Lecture 17
14.1. a.c. Stark effect (or light shift)
14.2. Dipole force and optical lattices.
14.2.1. Scattering rate.
14.2.2. Relationship between dipole force and light shift from a.c. Stark effect.
14.2.3. Sign of detuning determines direction of dipole force --> dipole trap.
14.2.4. Arrays of traps (lattices) formed from standing waves of laser light.
15. Time-dependence evolution of relative phase.
15.1. Absorption/emission of photon.
15.2. 2p0<->1s0 See movie 2p0to1s0.avi
15.2.1. Linear polarisation, pi-transition.
15.3. 2p1<->1s0 See movie 2p1to1s0.avi
15.3.1. Right circular polarisation, sigma^+ transition.
15.4. 2p-1<->1s0
15.4.1. Left circular polarisation, sigma^- transition.
15.5. 2p1<->1s0 See movie 2p1to1s0(2).avi
15.5.1. Horizontal linear polarisation, transverse observation.
16. Visualising electron distributions during transitions.
16.1. Foot §1.8 & §1.8,1 - Zeeman effect.
16.1.1. pi, sigma^+ & sigma^- polarisations.
17. Opposite parity. Electric dipole allowed transition.
18. Coupling between electromagnetism and gravity?
19. Compare electric and magnetic dipole interactions.
20. Why do energy levels split? Cont.
20.1. Calculate spin configuration state energies of H ground state using DPT.
20.1.1. Calculate eigenenergies.
20.1.2. Calculate eigenstates.
21. Lecture 1
21.1. History of clocks and timekeeping.
22. Lecture 2
22.1. How good is a clock?
22.1.1. Clock uncertainty.
22.1.2. Definition of SI second.
22.2. Principle of atomic clock.
22.2.1. Atomic transition provides reference frequency.
22.2.2. Advantages.
22.2.3. Interaction of atom with EM field.
22.2.4. Minimise broadening mechanisms.
22.3. How do atoms interact with EM fields?
22.3.1. Classical picture.
22.3.1.1. Electric dipole interaction.
22.3.1.2. Magnetic dipole interaction.
22.4. Electric dipole interactions.
22.4.1. Electric dipole moment.
22.4.2. Electric dipole approximation.
22.4.3. Does an atomic orbital have a dipole moment?
22.4.3.1. Expectation value of dipole moment operator.
22.4.3.1.1. Radial solutions.
22.4.4. Atkins §13.1 - The structure of hydrogenic atoms.
22.4.4.1. Separation of internal motion.
22.4.5. The structure of hydrogen and hydrogenic atoms.
22.4.5.1. Foot §2.1 - Schroedinger equation.
22.4.5.1.1. Solution of the angular equation.
22.4.5.1.2. Solution of the radial equation.
22.4.5.1.3. Energy levels.
22.4.5.2. Y&F §41.3 - Schroedinger equation for hydrogen atom.
22.4.5.2.1. Quantisation of orbital angular momentum.
22.4.5.2.2. Quantum number notation.
22.4.5.2.3. Electron probability distributions.
22.4.5.2.4. Hydrogenlike atoms.
22.4.5.3. Atkins §13.2 - Atomic orbitals and their energies.
22.4.5.3.1. Ionisation energies.
22.4.5.3.2. Shells and subshells.
22.4.5.3.3. Atomic orbitals.
22.4.5.3.4. Radial distribution functions.
22.4.5.3.5. p-orbitals.
22.4.5.3.6. d-orbitals.
22.4.6. Foot §2.2 (up to and inc. §2.2.1) - Transitions.
22.4.6.1. pi-transitions.
22.4.6.2. Selection rules.
22.4.6.2.1. sigma-transitions.
22.4.7. Does a superposition of eigenstates have a dipole moment?
22.4.7.1. Electric dipole moment associated with transitions between eigenstates.
22.4.8. Foot §2.2.3 - Parity.
22.5. Magnetic dipole interactions.
22.5.1. Y&F §41.4, p.1379 to 1382 - Zeeman effect.
22.5.1.1. Magnetic moment of orbiting electron.
22.5.1.2. Selection rules.
23. Lecture 3
23.1. Summary of electric dipole transitions
24. Lecture 4
24.1. Spontaneous emission.
24.1.1. Explicitly forbidden by quantum mechanics. Induced by vacuum fluctuations.
24.1.1.1. Vacuum fluctuations.
24.1.2. Foot §1.7 - Einstein A & B coefficients.
24.1.3. Hyperfine splitting.
24.1.4. Natural linewidth.
24.1.4.1. Rate of decay. Einstein A-coefficient and lifetime.
24.1.5. Calculate clock uncertainty of electric dipole allowed transition.
24.1.6. Calculate lifetime of H 2p state.
24.1.7. Is 2p<-1s in H a good clock transition?
24.2. Calculate spin-orbit splitting and energy shifts of fine structure states.
24.2.1. Quantised vector model.
24.3. How do we reduce Einstein A-coefficient?
24.3.1. Einstein A-coefficient for magnetic dipole allowed transition.
24.4. Why do energy levels split?
24.4.1. Foot §2.3 (excl. §2.3.4), also §2.1.2.
24.4.1.1. Fine structure / spin-orbit splitting.
24.4.1.1.1. Selection rules.
24.4.2. Foot §4.5 - The spin-orbit interaction: a quantum mechanical approach.
24.4.3. Foot §5 & §5.1 - The LS-coupling scheme.
24.4.3.1. Fine structure in the LS-coupling scheme.
24.4.4. Y&F §41.6 - Many electron atoms and the exclusion principle.
25. Lecture 5
25.1. Why do energy levels split? Cont.
25.1.1. Proton and electron magnetic moments.
25.1.2. Electron-proton spin-spin interaction hamiltonian.
25.1.3. Origin of hyperfine splitting.
25.1.3.1. Hyperfine splitting energy from 1st order TIPT.
25.1.4. Foot §6.1 (excl. §6.1.2 & §6.1.4) - Hyperfine structure.
25.1.5. Foot §6.1.3 - Hyperfine structure for l not equal to zero.
25.1.6. Calculate spin configuration state energies of H ground state using DPT.
26. Lecture 6
26.1. Effect on clock uncertainty.
26.1.1. Lifetime of spontaneous emission from upper hyperfine state.
26.1.2. Is H atom clock sensitive to external magnetic fields?
26.1.3. Foot Appendix C - Magnetic dipole transitions.
26.1.3.1. Summary of electric dipole and magnetic dipole transitions.
27. Lecture 18
27.1. 87Sr optical lattice clock
27.1.1. Laser cooling transition.
27.1.2. Clock transition - detection.
27.1.2.1. Doubly forbidden --> very narrow linewidth.
27.1.3. Magic wavelength.
27.1.4. Latest research - temperature stabilised clock / lattice in cryogenic environment.
27.2. Future of atomic clocks.
27.2.1. Are fundamental constants actually constant?
27.2.2. Special relativistic effects observed at very low speeds.
27.2.3. Relativistic geodesy.
27.2.3.1. Measure elasticity of Earth.
27.2.4. Nuclear clocks with 10^-19 fractional uncertainty.