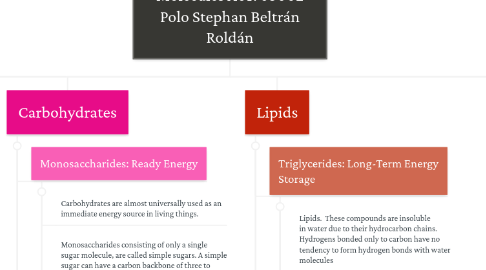
1. Organic molecules molecules that contain both carbon and hydrogen atoms
2. Organic Molecules
2.1. The Carbon Atom
2.1.1. Because carbon needs four electrons to complete its outer shell, it can share with as many as four other elements, and this spells diversity.
2.1.2. Hydrocarbons are chains of carbon atoms bonded exclusively to hydrogen atoms.
2.1.3. Carbon can form double bonds with itself and other atoms. Double bonds restrict the movement of bonded atoms, and in that way contribute to the shape of the molecule
2.2. The Carbon Skeleton and Functional Groups
2.2.1. The carbon chain of an organic molecule is called its skeleton or backbone.
2.2.2. So, the diversity of organic molecules comes about when different functional groups are added to the carbon skeleton. A functional group is a specific combination of bonded atoms that always reacts in the same way, regardless of the particular carbon skeleton.The attached functional groups determine the polarity of an organic molecule and also the types of reactions it will undergo.
2.2.3. hydrophobic (not soluble in water) hydrophilic (soluble in water)
2.2.4. Isomers are organic molecules that have identical molecular formulas but a different arrangement of atoms.
2.3. The Biomolecules of Cells
2.3.1. A cell uses a condensation reaction to synthesize (build up) any type of biomolecule. It’s called a dehydration reaction because the equivalent of a water molecule—that is, an OH (hydroxyl group) and an H (hydrogen atom), is removed as subunits are joined.
2.3.2. To break down biomolecules, a cell uses an opposite type of reaction. During a hydrolysis reaction, an JOH group from water attaches to one subunit, and an JH from water attaches to the other subunit.
2.3.3. Enzymes are required for cells to carry out dehydration and hydrolysis reactions. An enzyme is a molecule that speeds a reaction by bringing reactants together, and the enzyme may even participate in the reaction but it is unchanged by it.
2.3.4. The largest of the biomolecules are called polymers and like all biomolecules, polymers are constructed by linking together a large number of the same type of subunit. However, in the case of polymers, the subunits are called monomers. A polysaccharide, a protein and a nucleic acid, is a polymer that contains innumerable monomers.
3. Carbohydrates
3.1. Monosaccharides: Ready Energy
3.1.1. Carbohydrates are almost universally used as an immediate energy source in living things.
3.1.2. Monosaccharides consisting of only a single sugar molecule, are called simple sugars. A simple sugar can have a carbon backbone of three to seven carbons.
3.1.3. Glucose, with six carbon atoms, is a hexose and has a molecular formula of C6H12O6. This simple sugar is the major source of cellular fuel for all living things. Glucose is transported in the blood of animals, and it is the molecule that is broken down in nearly all types of organisms during cellular respiration, with the resulting buildup of ATP molecules.
3.2. Disaccharides: Varied Uses
3.2.1. A disaccharide contains two monosaccharides that have joined during a dehydration reaction.
3.2.2. Sucrose (the structure shown at right) is another disaccharide of special interest because it is sugar we use at home to sweeten our food.
3.3. Polysaccharides: Energy Storage Molecules
3.3.1. Polysaccharides are polymers of monosaccharides. Some types of polysaccharides function as short-term energy storage molecules. When an organism requires energy, the polysaccharide is broken down to release sugar molecules.
3.3.2. Plants store glucose as starch. The cells of a potato contain granules where starch resides during winter until energy is needed for growth in the spring
3.3.3. Animals store glucose as glycogen. In our bodies and those of other vertebrates, liver cells contain granules where glycogen is being stored until needed. The storage and release of glucose from liver cells is under the control of hormones. After we eat, the release of the hormone insulin from the pancreas promotes the storage of glucose as glycogen
3.3.4. Polysaccharides serve as storage molecules because they are not as soluble in water, and are much larger than a sugar. Therefore, polysaccharides cannot easily pass through the plasma membrane, a sheetlike structure that encloses cells.
3.4. Polysaccharides: Structural Molecules
3.4.1. Structural polysaccharides include cellulose in plants, chitin in animals and fungi, and peptidoglycan in bacteria
3.4.2. Cellulose is the most abundant carbohydrate and, indeed, the most abundant organic molecule on Earth
3.4.3. Chitin is found in fungal cell walls and in the exoskeletons of crabs and related animals, such as lobsters, scorpions, and insects. Chitin, like cellulose, cannot be digested by animals; however, humans have found many other good uses for chitin.
4. Lipids
4.1. Triglycerides: Long-Term Energy Storage
4.1.1. Lipids. These compounds are insoluble in water due to their hydrocarbon chains. Hydrogens bonded only to carbon have no tendency to form hydrogen bonds with water molecules
4.1.2. Fats and oils contain two types of subunit molecules: fatty acids and glycerol. Each fatty acid consists of a long hydrocarbon chain with a COOH (carboxyl) group at one end. Fatty acids are either saturated or unsaturated. Saturated fatty acids have no double bonds between the carbon atoms. The carbon chain is saturated, so to speak, with all the hydrogens that can be held. Unsaturated fatty acids have double bonds in the carbon chain wherever the number of hydrogens is less than two per carbon atom.
4.1.3. Glycerol is a compound with three OH groups. The OH groups are polar; therefore, glycerol is soluble in water. When a fat or oil forms, the acid portions of three fatty acids react with the OH groups of glycerol during a dehydration reaction
4.1.4. Fats and oils are degraded following a hydrolysis reaction. Because there are three fatty acids attached to each glycerol molecule, fats and oils are sometimes called triglycerides
4.2. Phospholipids: Membrane Components
4.2.1. Phospholipids, as implied by their name, contain a phosphate group.
4.2.2. A phospholipid is constructed like a fat, except that in place of the third fatty acid attached to glycerol, there is a polar phosphate group.
4.3. Steroids: Four Fused Rings
4.3.1. Steroids are lipids that have entirely different structures from those of fats. Steroid molecules have skeletons of four fused carbon rings.
4.3.2. Cholesterol is an essential component of an animal cell’s plasma membrane, where it provides physical stability. Cholesterol is the precursor of several other steroids, such as the sex hormones testosterone and estrogen.
4.4. Waxes
4.4.1. In waxes, long-chain fatty acids bond with long-chain alcohols.
4.4.2. Waxes are solid at normal temperatures because they have a high melting point. Being hydrophobic, they are also waterproof and resistant to degradation.
5. Proteins
5.1. Proteins
5.1.1. Proteins are such a major part of living organisms that tissues and cells of the body can sometimes be characterized by the proteins they contain or produce.
5.2. Peptides
5.2.1. The resulting covalent bond between two amino acids is called a peptide bond. The atoms associated with the peptide bond share the electrons unevenly because oxygen is more electronegative than nitrogen.
5.2.2. A peptide is two or more amino acids bonded together, and a polypeptide is a chain of many amino acids joined by peptide bonds. A protein may contain more than one polypeptide chain; therefore, you can see why a protein could have a very large number of amino acids.
5.3. Amino Acids: Protein Monomers
5.3.1. The name amino acid is appropriate because one of these groups is an NH2 (amino group) and another is a COOH (an acid group). The third group is called an R group for an amino acid.
5.4. Shape of Proteins
5.4.1. A protein can have up to four levels of structure, but not all proteins have all four levels.
5.4.2. The primary structure of one protein is its own particular sequence of amino acids.
5.4.3. The secondary structure of a protein occurs when the polypeptide coils or folds in a particular way.
5.4.3.1. Fibrous proteins, which are structural proteins, exist as helices or pleated sheets that hydrogen-bond to each other. Examples are keratin, a protein in hair and silk, a protein that forms spider webs. Both of these proteins have only a secondary structure
5.4.4. A tertiary structure is the folding that results in the final threedimensional shape of a polypeptide. So-called globular proteins, which tend to ball up into rounded shapes, have a tertiary structure. Hydrogen bonds, ionic bonds, and covalent bonds between R groups all contribute to the tertiary structure of a polypeptide.
5.4.5. Some proteins have a quaternary structure because they consist of more than one polypeptide. Hemoglobin is a much-studied globular protein that consists of four polypeptides, and therefore it has a quaternary structure. Each polypeptide in hemoglobin has a primary, secondary, and tertiary structure.
5.5. Protein-Folding Diseases
5.5.1. Proteins cannot function properly unless they fold into their correct shape. In recent years it has been shown that the cell contains chaperone proteins, which help new proteins fold into their normal shape.
6. Nucleic Acids
6.1. Nucleic acids are polymers of nucleotides with very specific functions in cells. DNA (deoxyribonucleic acid) is the genetic material that stores information regarding its own replication and the order in which amino acids are to be joined to make a protein. RNA (ribonucleic acid) is another type of nucleic acid. One type of RNA molecule called messenger RNA (mRNA) is an intermediary in the process of protein synthesis, conveying information from DNA regarding the amino acid sequence in a protein.
6.2. Structure of DNA and RNA
6.2.1. Every nucleotide is a molecular complex of three types of molecules: phosphate (phosphoric acid), a pentose sugar, and a nitrogen-containing base
6.2.2. There are four types of nucleotides in DNA and four types of nucleotides in RNA.
6.2.3. Nucleotides join in a definite sequence by a series of dehydration reactions when DNA and RNA form. The polynucleotide is a linear molecule called a strand in which the backbone is made up of a series of sugar-phosphate-sugarphosphate molecules. The bases project to one side of the backbone. Since the nucleotides occur in a definite order, so do the bases. RNA is single stranded.
6.2.4. DNA is double stranded, with the two strands usually twisted about each other in the form of a double helix. The two strands are held together by hydrogen bonds between pyrimidine and purine bases.
6.2.5. The bases can be in any order within a strand, but between strands, thymine (T) is always paired with adenine (A), and guanine (G) is always paired with cytosine (C). This is called complementary base pairing.
6.3. ATP (Adenosine Triphosphate)
6.3.1. ATP is a nucleotide in which adenosine is composed of adenine and ribose. Triphosphate stands for the three phosphate groups that are attached together and to ribose, the pentose sugar
6.3.2. The energy that is released by ATP breakdown is coupled to energy-requiring processes in cells.