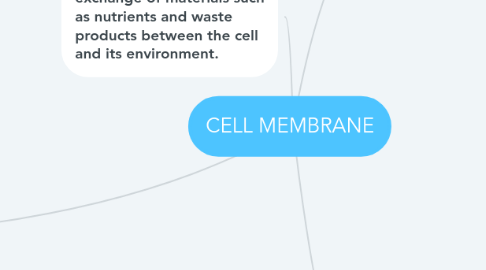
1. FUNCTION : controls the exchange of materials such as nutrients and waste products between the cell and its environment.
2. Functions of the components
2.1. Phospholipids
2.1.1. Phospholipids can be modified chemically to act as signalling molecules. They may move about in thephospholipid bilayer, activating other molecules such as enzymes. Alternatively, they may be hydrolysed to release small, water-soluble, glycerol-related molecules. These diffuse through the cytoplasm and bind to specific receptors.
2.2. Cholesterol
2.2.1. Cholesterol is a relatively small molecule. Like phospholipids, cholesterol molecules have hydrophilic heads and hydrophobic tails, so they fit neatly between the phospholipid molecules with their heads at the membrane surface. Cell surface membranes in animal cells contain almost as much cholesterol as phospholipid.
2.2.1.1. At low temperatures, cholesterol increases the fluidity of the membrane, preventing it from becoming too rigid. This is because it prevents close packing of the phospholipid tails. The increased fluidity means cells can survive colder temperatures.
2.2.1.2. The interaction of the phospholipid tails with the cholesterol molecules also helps to stabilise cells at higher temperatures when the membrane could otherwise become too fluid.
2.2.2. Cholesterol is also important for the mechanical stability of membranes, as without it membranes quickly break and cells burst open. The hydrophobic regions of cholesterol molecules help to prevent ions or polar molecules from passing through the membrane. This is particularly important in the myelin sheath (made up of many layers of cell surface membrane) around nerve cells, where leakage of ions would slow down nerve impulses.
2.3. Glycolipids, glycoproteins and proteins
2.3.1. Many of the lipid molecules on the outer surfaces of cell surface membranes, and probably all of the protein molecules, have short carbohydrate chains attached to them. These ‘combination’ molecules are known as glycolipids and glycoproteins, respectively.
2.3.2. The carbohydrate chains project like antennae into the watery fluids surrounding the cell, where they form hydrogen bonds with the water molecules and so help to stabilise the membrane structure. The carbohydrate chains form a sugary coating to the cell, known as the glycocalyx.
2.3.2.1. In animal cells, the glycocalyx is formed mainly from glycoproteins.
2.3.2.2. In plant cells it mainly comprises glycolipids.
2.3.2.3. The carbohydrate chains help the glycoproteins and glycolipids to act as receptor molecules, which bind with particular substances at the cell surface. Different cells have different receptors, depending on their function. There are three major groups of receptor.
2.3.2.3.1. One group of receptors can be called ‘signalling receptors’, because they are part of a signalling system that coordinates the activities of cells. The receptors recognise messenger molecules like hormones and neurotransmitters. When the messenger molecule binds to the receptor, a series of chemical reactions is triggered inside the cell.
2.3.2.3.2. A second group of receptors are involved in endocytosis . They bind to molecules that are parts of the structures to be engulfed by the cell surface membrane.
2.3.2.3.3. A third group of receptors is involved in binding cells to other cells (cell adhesion) in tissues and organs of animals.
2.3.3. Some glycolipids and glycoproteins act as cell markers or antigens, allowing cell–cell recognition. Each type of cell has its own type of antigen, rather like countries with different flags. For example, the ABO blood group antigens are glycolipids and glycoproteins which have small differences in their carbohydrate portions.
2.3.4. Many proteins act as transport proteins. These provide hydrophilic channels or passageways for ions and polar molecules to pass through the membrane. There are two types of transport protein: channel proteins and carrier proteins. . Each transport protein is specific for a particular kind of ion or molecule. Therefore the types of substances that enter or leave the cell can be controlled.
2.4. Other proteins
2.4.1. Other membrane proteins may be enzymes – for example, the digestive enzymes found in the cell surface membranes of the cells lining the small intestine. These catalyse the hydrolysis of molecules such as disaccharides.
2.4.2. Some proteins on the inside of the cell surface membrane are attached to a system of protein filaments inside the cell, known as the cytoskeleton. These proteins help to maintain and decide the shape of the cell. They may also be involved in changes of shape when cells move.
2.4.3. Proteins also play important roles in the membranes of organelles.
3. In 1972, two scientists, Singer and Nicolson, used all the available evidence to put forward a hypothesis for membrane structure. They called their model the fluid mosaic model.
3.1. It is described as ‘fluid’ because both the phospholipids and the proteins can move about by diffusion. The word ‘mosaic’ describes the pattern produced by the scattered protein molecules when the surface of the membrane is viewed from above.
4. Features & of components of the fluid mosaic model
4.1. The membrane is a double layer (bilayer) of phospholipid molecules. The individual phospholipid molecules move about by diffusion within their own monolayers.
4.2. The phospholipid tails point inwards, facing each other and forming a non-polar hydrophobic interior. The phospholipid heads face the aqueous (water-containing) medium that surrounds the membranes.
4.3. Some of the phospholipid tails are saturated and some are unsaturated. The more unsaturated they are, the more fluid the membrane. This is because the unsaturated fatty acid tails are bent and therefore fit together more loosely.
4.3.1. Fluidity is also affected by tail length: the longer the tail, the less fluid the membrane.
4.4. As temperature decreases, membranes become less fluid
4.4.1. some organisms which cannot regulate their own temperature, such as bacteria and yeasts, respond by increasing the proportion of unsaturated fatty acids in their membranes.
4.5. Two types of protein are recognised, according to their position in the membrane.
4.5.1. Proteins that are found embedded within the Membrane are called intrinsic proteins (or integral proteins).
4.5.1.1. Intrinsic proteins may be found in the inner layer, the outer layer or, most commonly, spanning the whole membrane, in which case they are known as transmembrane proteins.
4.5.1.1.1. In transmembrane proteins, the hydrophobic regions which cross the membrane are often made up of one or more α-helical chains.
4.5.1.2. Intrinsic proteins have hydrophobic and hydrophilic regions.
4.5.1.3. They stay in the membrane because the hydrophobic regions, made from hydrophobic amino acids, are next to the hydrophobic fatty acid tails and are repelled by the watery environment either side of the membrane. The hydrophilic regions, made from hydrophilic amino acids, are repelled by the hydrophobic interior of the membrane and therefore face into the aqueous environment inside or outside the cell, or line hydrophilic pores which pass through the membrane.
4.5.1.4. Most of the intrinsic protein molecules float like mobile icebergs in the phospholipid layers, although some are fixed like islands to structures inside or outside the cell and do not move about.
4.5.2. A second type of protein molecule is the extrinsic protein (or peripheral protein).
4.5.2.1. These are found on the inner or outer surface of the membrane. Many are bound to intrinsic proteins. Some are held in other ways – for example, by binding to molecules inside or outside the cell, or to the phospholipids.
4.5.2.2. Many proteins and lipids have short, branching carbohydrate chains attached to that side of the molecule which faces the outside of the membrane, thus forming glycoproteins and glycolipids, respectively. The total thickness of the membrane is about 7nm on average. Molecules of cholesterol are also found in the membrane.