Energy Conversion Efficiency Mechanisms In Quantum Dot Intermediate Band Nanostructure Solar Cells
by jose diaz
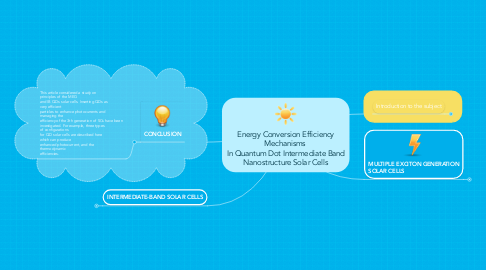
1. CONCLUSION
1.1. This article considered a study on principles of the MEG and IB QDs solar cells. Inserting QDs as very efficient particles to enhance photocurrents and managing the efficiency of the 3th generation of SCs have been investigated. For example, three types of configurations for QD solar cells are described here which can produce enhanced photocurrent, and the thermodynamic efficiencies.
2. Introduction to the subject
2.1. We have reviewed the application of quantization effects in nanocrystal particles to produce third-generation Quantum Dot (QD) Solar Cells (SC) that leads to very low cost solar electricity. We discuss two roads based on semiconductor QDs and QDs arrays that will lead to ultrahigh efficiencies through enhanced photocurrent.
3. INTERMEDIATE-BAND SOLAR CELLS
3.1. To capture and use photons which are less than the bandgap energy, IB solar cells are based on intermediate band materials
3.1.1. These materials are characterized by the existence of an intermediate band located between the conventional semiconductor conduction band (CB) and valence band (VB) Due to the IB, photons with energy below the bandgap can contribute to the cell photocurrent by exciting electrons from the VB to the IB and from the IB to the CB.