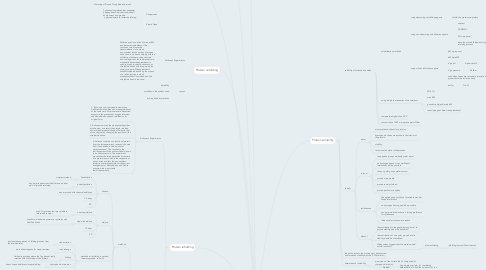
1. Protein unfolding
1.1. Anfinsen's Experiments
1.1.1. Anfinsen used a solution of urea at 8M and beta-mercaptoethanol. The function of urea in protein denaturalization is to break non-covalent bonds such as hydrogen bonds and ionic bonds helping with the unfolding of the secondary structure and some parts of the tertiary structure, meanwhile beta-mercaptoethanol is used to break covalent bonds such as disulphide bonds unfolding mainly the tertiary structure. Mercaptoethanol breaks disulphide bonds by the means of a redox reaction in which mercaptoethanol is oxidized and the disulphide bond is reduced.
1.2. causes
1.2.1. instability
1.2.2. solubility in the solvent used
1.2.3. hydrophobic interactions
2. Protein refolding
2.1. Anfinsen's Experiments
2.1.1. 1- By using a semi-permeable membrane Anfinsen removed urea and mercaptoethanol at the same time. After some time denatured enzyme in the presence of oxygen reformed the disulphide bonds and refolded to its original form.
2.1.2. 2- Anfinsen removed the mercaptoethanol but not the urea, as a result the protein refolded into a three-dimetional shape different to that of the original, by changing the position of the disulphide bonds.
2.1.3. 3- Anfinsen took the non-functional protein from the last experiment, removed the urea from it and added a little amount of mecaptoethanol. This resulted in the rearrangement of the non-functional protein to its native structure. This experiment proved that the three-dimensional structure of a protein is encoded in the sequence of amino acids and that the non-covalent interactions are responsible for the correct arrangement of disulphide bonds. Native enzyme is the most stable thermodynamically.
2.2. refolding og nclusion bodies
2.2.1. methods
2.2.1.1. dilution
2.2.1.1.1. flash dilution
2.2.1.1.2. pulsatile dilution
2.2.1.1.3. also improved with chemical additives
2.2.1.1.4. 1-2 days
2.2.1.1.5. 4°C
2.2.1.2. dialysis
2.2.1.2.1. one step dialysis
2.2.1.2.2. step wise dialysis
2.2.1.2.3. 1-2 days
2.2.1.2.4. 4°C
2.2.1.3. methods of refolding in packed chromatographic columns
2.2.1.3.1. size exclusion
2.2.1.3.2. ion exchange
2.2.1.3.3. affinity
2.2.1.3.4. hydrophobic interaction
2.2.1.3.5. in presence of chaperons
2.2.1.4. microfluidic chips
2.2.1.4.1. used with difficult to fold proteins
2.2.1.4.2. recovery yield 70%
2.2.1.4.3. 10 min
2.2.1.4.4. 25°C
2.2.1.5. urease mediated refolding
2.2.1.5.1. using the refolding buffer is not necessary because the enzyme is responsible of the gradual removal of the urea used to solubilize the protein
2.2.2. buffer/additives
2.2.2.1. urea (2M)
2.2.2.2. dodecyl maltoside (5mM)
2.2.2.3. n.pentanol (5mM)
2.2.2.4. ammonium sulfate (0.5M)
2.2.2.5. guanidine hydrochloride (1M)
2.2.2.6. arginine (0.25M)
2.2.2.7. proline (1M)
2.2.2.8. sucrose (0.5M)
2.2.2.9. polyethylene glycol (0.05%)
2.2.2.10. glycerol (0.5M)
2.2.2.11. cysteine or cystine (5-15 mM)
2.2.3. concentration of the protein
2.2.3.1. 10 to 50 micrograms pero milliliter
3. Roles of enzymes
3.1. In biotechnology
3.1.1. Molecular biology
3.1.1.1. Metabolic pathways
3.1.2. Bioprocesses
3.1.2.1. Inmoilized enzyme bioreactor
3.1.2.2. Tunable multi-enzyme-coordinate expression system: Multi-enzymatic cascade reactions, these consecutive reactions offer considerable advantages like decreasing the amount of time for a process to finish, chemical processes have low chiral selectivity while enzymes are very specific, etc.
3.2. In modern society
3.2.1. New materials
3.2.1.1. Graffting
3.2.2. Cosmeceutical
3.2.2.1. Enzyme Peeling
4. Protein folding
4.1. Folding funnels
4.1.1. Energy Landscape: Is a funnel like diagram in which a protein can be described by the free energy of the polypeptide chain and conformation.
4.2. Levinthian paradox
4.2.1. Proteins are super complex molecules and in order to find the natural folded structure of one by random mathematical search would take billions of years, but in nature proteins fold within seconds
4.3. Chaperones
4.3.1. Molecular machinery: Proteins that assist the folding process of nascent chains from the ribosome; help to reach the native state of a protein but do not participate in its final structure and function.
4.4. Anfinsen's Dogma
4.4.1. The three-dimensional structure of a native protein in its normal physiological milieu (pH, solvent, temperature, etc.) is the one in which the Gibbs free energy of the whole system is lowest.
4.4.2. Discovery of Protein Disulphide Isomerase.
4.5. Chaperonins
4.5.1. Cylindrical complexes that sequester polypeptides from the crowded cell environment and provide a physical space for effective folding.
4.6. Kinetic Traps
5. Protein Structure
5.1. Primary Structure
5.1.1. Amino acids
5.1.1.1. Polar
5.1.1.1.1. Acid
5.1.1.1.2. Basic
5.1.1.1.3. Uncharged side chain
5.1.1.2. Non-polar
5.1.1.2.1. Alanine (Ala,A)
5.1.1.2.2. Glycine (Gly,G)
5.1.1.2.3. Leucine (Leu,L)
5.1.1.2.4. Isoleucine (Ile, I)
5.1.1.2.5. Valine (Val, V)
5.1.1.2.6. Methionine (Met,M)
5.1.1.2.7. Proline (Pro,P)
5.1.1.2.8. Phenyllalanine (Phe,F)
5.1.1.2.9. Tryptophan (Trp, W)
5.2. Secondary Structure
5.2.1. alpha helix
5.2.2. beta sheets
5.3. Tertiary Structure
5.3.1. protein domains
5.3.2. one or more secondary structure
5.4. Quaternary Structure
5.4.1. one or more polypeptide chain
5.4.2. two or more subunits
6. Protein Stability
6.1. Folding funnels have a tendecy to have the native form of the protein as the lowest free energy, at this point its stability is the highest, this has been achieved because of evolution
6.2. consequences if not stable
6.2.1. incorrect folding
6.2.1.1. formation of inclusion bodies
6.2.1.1.1. causes
6.2.2. unfolding
6.3. depends on
6.3.1. 3D strucutre
6.3.2. hydrophobic interactions
6.3.3. solvent
6.4. forces stabilizing proteins
6.4.1. conformation entropy
6.4.1.1. major force destabilizing proteins
6.4.1.2. rotation around the bonds
6.4.2. charge charge interactions and salt bridges
6.4.2.1. charges in the surface generally arranged so that there are more attractive than repulsive interactions near neutral pH
6.4.2.2. electrostatic interactions contribute favourably
6.4.3. hydrophobic interaction
6.4.3.1. dominant force of protein folding
6.4.4. hydrogen bonding and polar group burial
6.4.4.1. generally contribute +1kcal/mol per hydrogen bond to the energetics of substrate binding
7. Protein solubility
7.1. solubility of Inclussion boddies
7.1.1. solubilization methods
7.1.1.1. using denaturing solubilizing agents
7.1.1.1.1. unfold the protein completely
7.1.1.2. using non-denaturing solubilization agents
7.1.1.2.1. sarcosyl
7.1.1.2.2. 5% DMSO
7.1.1.2.3. 5% n-propanol
7.1.1.2.4. leave the protein folded and toy do not need a refolding process
7.1.1.3. using mild solubilization agents
7.1.1.3.1. 6M n-propanol
7.1.1.3.2. 6M beta ME
7.1.1.3.3. high pH
7.1.1.3.4. high pressure
7.1.1.3.5. used when the native secondary structure is preserved in the inclusion body
7.1.1.3.6. buffer
7.1.2. using a high concentration of denaturants
7.1.2.1. SDS 1%
7.1.2.2. urea 8M
7.1.2.3. guanidine hydrochloride 6M
7.1.2.4. reducing agent: beta mercaptoethanol
7.1.3. temperature higher than 30°C
7.1.4. concentration 10-50 micrograms per milliliter
7.2. solvent
7.2.1. water
7.2.1.1. most preferred solvent for proteins
7.2.1.2. hydrophobic chains are apcked in the interior of the protein
7.2.1.3. stability
7.2.1.4. favours native state of the proteins
7.2.2. vacuum
7.2.2.1. the peptide groups markedly prefer water
7.2.2.2. no hydrogen bonds or van der Waals interactions will be possible
7.2.2.3. the non polar goups prefer vacuum
7.2.2.4. protein more stable
7.2.2.5. protein remains folded
7.2.2.6. protein packs more tightly
7.2.3. cyclohexane
7.2.3.1. The eptide goups will have favourable wan der Waals interactions
7.2.3.2. no hydrogen boding would be possible
7.2.3.3. non-polar side chains have a strong preference for cyclohexane
7.2.3.4. folded conformation more stable
7.2.4. ethanol
7.2.4.1. the contribution to the peptide group burial to protein stability would be enhanced
7.2.4.2. the contribution of non-polar group burial to stability would be diminished
7.2.4.3. Gibbs values suggest that the protein would unfold in ethanol
7.2.4.3.1. after unfolding
7.3. the native state fo the protein must be favoured (conformation stability must be 2-10 kcal/mol)
7.4. determinant of solubility
7.4.1. proportion of the surface that is composed of charged side chains
8. Protein identification
8.1. Total Nitrogen Methods
8.1.1. Kjeldahl
8.1.1.1. Very simple principle, for cuantitative determination to find the amount of N in a sample
8.1.2. Dumas
8.1.2.1. More complex process, but fast and precise for cuantification on N in a sample
8.2. Chromatography
8.2.1. Thin layer
8.2.2. Gas
8.2.3. Liquid
8.2.3.1. HPLC
8.2.3.1.1. The components of the sample mixture are separated from each other due to their different degrees of interaction with the absorbent particles.
8.2.4. Supercritical fluid
8.2.5. ion exchange
8.2.6. Hydrophobic interaction
8.2.7. Two-dimesional
9. Protein analysis
9.1. Spectrophotometic Methods
9.1.1. UV absorption
9.1.1.1. Measured at @280nm thanks to aromaticity of some aminoacid residues
9.1.1.2. Fast, non destructive and flexible, but nucleic acids can interfeer
9.1.2. Bradford
9.1.3. Bincinchoninic acid
9.1.3.1. A compound capable of forming a purple complex with cuprous ion Cu+1 in an alkaline environment. This reagent forms the basis of an analytical method capable of monitoring cuprous ion produced in the reaction of protein with alkaline Cu2+ (biuret reaction).
9.1.3.2. Read at 562nm and has a detection range that goes from 0.2 to 50 micrometers.
9.1.4. Ninhydrin
9.1.4.1. A compound that reacts with amino acids and turns deep purple. Ninhydrin will react with a free alpha-amino group, not with proteins nor peptides.
9.1.4.2. Measured at 570nm for amino acids and 440nm in imino acids.
9.1.5. Infrared absorption
9.1.5.1. For a molecule to absorb IR, the vibrations or rotations within a particle must cause a net change in the dipole moment. If the frequency of the radiation matches the vibrational frequency of the molecule then there will be a change in the amplitude of molecular vibration.
9.1.5.2. The most useful wavenumber range in IR absorption lies between 4000-670cm^-1.
9.2. Sequential and Structural Techniques
9.2.1. Edman degradation
9.2.1.1. It is a cyclic degradation of peptides based on the reaction of phenylisothiocynate with the free amino group of the N-terminal residue.
9.2.1.1.1. amino acids are removed one at a time and identified
9.2.1.2. Steps:
9.2.1.2.1. 1) if it has 1+ chains = separate them
9.2.1.2.2. 2) cleave disulfide bonds (with performic acid)
9.2.1.2.3. 3) determine a. a. composition
9.2.1.2.4. 4) indentify N- and C- terminal
9.2.1.2.5. 5) cleave into smaller fragments and determine the sequence
9.2.1.2.6. 6) repeat step 6 with a different cleavage pattern
9.2.1.2.7. 7) with this information reconstruct the sequence
9.2.1.2.8. 8) locate disulfide bonds positions
9.2.1.3. advantages:
9.2.1.3.1. yield close to 100%
9.2.1.4. disadvantages
9.2.1.4.1. maximal length of the sequence 50aa
9.2.1.5. limitations
9.2.1.5.1. glycosilated and phosporylated aa may result in blank cycles or weird results
9.2.2. X-Ray crystallography
9.2.2.1. Crystals should have a high degree of order in their structure.
9.2.2.1.1. Crystals should also have a high level of purity
9.2.2.2. Protein crystallization Optical setup Diffraction analysis Data collection Data processing Determination of the amplitudes Determination of the phase Calculation of electron density map Refinement and model building
9.2.2.3. advantages
9.2.2.3.1. gives a very accurate 3D structure of the protein
9.2.2.4. disadvantages
9.2.2.4.1. expensive
9.2.2.4.2. diffraction image becomes weaker at high resolution
9.2.2.5. limitations
9.2.2.5.1. growth of protein crystals of sufficient quality
9.2.2.5.2. glycosilated or other type or special proteins are difficult to crystalize
9.2.3. Nuclear magnetic resonance
9.2.3.1. it uses the magnetic field to change the spin of the electrons of O, N and H atoms. That releases energy and according to its intensity or position, an assumption of the composition and structure of the sample is made
9.2.3.2. disadvantages
9.2.3.2.1. very expensive
9.2.3.2.2. relatively low analytical sensitivity
9.2.3.3. limitations
9.2.3.3.1. complex samples are difficult to asses
9.2.3.4. advantages
9.2.3.4.1. it is very precise, you can have a very clear image of the 3D structure